Integrated biological effects and chemical contaminants approach: a case study
1. Introduction
Diffuse chemical contamination in the ocean induces biological effects that impact the health of marine organisms and biodiversity. The major concerns related to chemical pollution are that some contaminants may be so persistent that they will remain in the marine environment for a very long time, even for centuries (Hylland et al., 2017a). Chemicals may also accumulate in organisms and/or in food chains up to concentrations leading to direct or indirect effects, including combined insidious effects at very low concentrations. Other chemical contaminants that are not very persistent but very labile may also impact organisms at early stages of development and throughout their life cycle. The major monitoring challenge is to assess the combined effects of approximately 140 000 chemicals including persistent or fleeting contaminants on organisms when less than 1% of chemical contaminant families are routinely measured.
Mixtures of naturally occurring and human-made contaminants are responsible for many of the toxicity mechanisms now observable in monitoring such as carcinogenicity, genotoxicity, neurotoxicity, immunotoxicity, cardiotoxicity, endocrine disruption, reprotoxicity and developmental toxicity (ICES, 2021). All these toxic mechanisms define the biological effects of the chemical contaminants. Although other environmental factors may interact with these toxic mechanisms, the cell remains the key to the entry of chemical molecules into biological tissues. Exposure to chronic and diffuse chemical stress induces toxicity mechanisms and then promotes alteration of major biological functions such as growth, reproduction, neurological behaviour, immune responses and disease over the medium to long term.
Ten years ago, the ICES Working Group on Biological Effects of Chemical Contaminants (WGBEC) developed a methodology to assess biological effects related to chemical exposure in sentinel species, sediment and water (ICES, 2011). In parallel, quality assurance activities have been established to ensure consistency in effect data from national and international monitoring programmes in the OSPAR area. Many scientific barriers inherent in biology, chemistry and more generally in oceanography are still to be identified to distinguish the biological effects of chemical contaminants and environmental factors. Nevertheless, the interest of this pioneer methodology to characterise the biological effect of the chemical contamination has been co-validated by scientists from eleven OSPAR Contracting Parties (Davies & Vethaak, 2012) and is now applied by several OSPAR countries and referred to as the ‘integrated approach’. The adoption of this approach into the Coordinated Environmental Monitoring Programme (CEMP) would greatly optimise the acquisition of new biological data. It would also provide a spatiotemporal biomonitoring of the health of the organisms exposed to contaminant mixtures and meets the technical impossibility of measuring the 140 000 chemical molecules that contaminate the oceans and grow faster than the world’s population (Judson et al., 2009; Roose et al., 2011).
The biological and chemical integrated approach is based on several structuring points:
- The selection of twenty marine biomarkers and bioassays developed on marine sentinel species and marine sediments for which (i) analytical reference methods for bioassays and biomarkers (ICES TIMES references) have been developed, (ii) quality assurance has been provided and (iii) thresholds for common interpretation in biota and sediment have been studied (ICES 2011, Davies and Vethaak, 2012). Effect thresholds (EAC: Environment Assessment Criteria) and baseline levels (BAC: Background Assessment Concentration) for biomarkers and bioassays have been established for the first time from ICES field data acquired using the same analytical methods and sampling strategies at contrasting sites in the coastal and offshore OSPAR areas. They have been validated in fish, gastropods and mussels sentinel species on a large geographical scale in the North-East Atlantic area between Iceland and Spain (ICON Project, Hylland et al., 2017b).
- A pre-CEMP voluntary approach developed with a common monitoring anchor integrating chemical guidelines of organic and metallic contaminants already measured in the CEMP.
- Common application of an optimised integrated approach chemistry-biology with colour codes harmonised according to Davies & Vethaak (2012) by eleven Contracting Parties participating to this chapter.
- Adoption of a common MIME spreadsheet for identical data processing (MIME 2019).
Different scenarios of biological effect measurements are described by eleven Contracting parties and discussed at a regional scale (section 2) and local/national scale including short-term (section 3) and long-term (section 4) time series. The various case studies presented in this chapter confirm the research dynamics of eleven OSPAR Contracting Parties to address the major challenge of monitoring the biological effects of contaminants. They also highlight how different regional initiatives (new sentinel species - or long-term sampling strategy on contaminated or pristine sites) enhance an integrated approach to monitoring the biological effects of chemical contaminants.
2. Biological effects of chemical contaminants on a broad geographical scale
Several thousand tons of waste including hazardous substances are discharged every minute into European marine waters (Hylland et al., 2017b). It is crucial to (i) understand how organisms cope with chemical exposure stress in a context of global change and (ii) assess the biological effects of chemical mixtures on organisms and populations living in a large geographic area such as the North-East Atlantic.
Oceanographic monitoring programmes are developed from the collection of numerous data on water, sediment and biota matrices, which requires an aggregation approach before restitution and use by regulatory decisions. In 2008-2012, several countries (Norway, France, England, Scotland, Ireland, Spain, Denmark, Germany, Iceland and the Netherlands) have validated an integrated approach to the biological effects of chemical contaminants in the marine environment based on harmonized oceanographic samplings (2008-2009), so called, the Integrated Assessment of Contaminant Impacts on the North Sea (ICON, Hylland et al., 2017b). Several of these countries also have oceanographic facades in the Baltic and Mediterranean Sea and therefore sampling sites covered by the ICON project include Iceland, North Sea, Baltic Sea, Wadden Sea, Seine estuary and western Mediterranean Sea. The methodology used in the present chapter is directly inspired by the ICON project and the integrated chemical and biological approach developed by ICES experts working in a special study group (SGIMC, 2011), both published in a collective report (Davies & Vethaak, 2012). This approach, developed on the basis of OSPAR/ICES field data, satisfies the need to go toward ecosystemic approaches in environmental assessments for data integration and aggregation in the Marine Strategy Framework Directive (MSFD). It incorporates contaminant and biological effect monitoring data and allows assessments to be made across matrices, sites and regions, together with multiple levels of aggregation for different assessment requirements. Early developments on biological effects were encouraged for the OSPAR QSR 2010 (OSPAR Commission 2009) and can be extended to include measurement of other chemical and biological effects. Threshold criteria are used to identify responses above context (BAC: Background Assessment Concentration) and responses that indicate probable impacts at the population level and concentration of chemical above EAC. This approach also addresses GES (Good environmental status) assessment as required by descriptor 8 of the MSFD ("Concentrations of contaminants are at levels not giving rise to pollution effects").
An excel spreadsheet validated by MIME 2019 makes it possible to harmonise the compilation of chemical and biological data and to standardise the calculation of the four aggregation steps of the Davies & Vethaak method (2012). The assessment criteria used for the current integrated assessment are summarised in Table 1. The co-authors provided chemical data and biological effects data for each station on different fish, gastropods, mussels and sediments (Table 2). Initial step 1 is the assessment of each parameter in each matrix at each station regarding chemical concentrations or biological responses with a traffic light approach (less than BAC (Blue), between the BAC and EAC (green) or above EAC (red)). The three following steps enable to integrate the parameters by matrix at each site (step 2), across matrix at each site (step 3) and finally across sites (step 4).
Step 1 : Assessment of monitoring data matrix against BAC and EAC
Sediment | |
---|---|
Determinand | Status |
Cadmium | |
Mercury | |
Lead | |
Copper | |
Zinc | |
PCB | |
BFR | |
TBT | |
Arenicola bioassay | |
Sea urchin extract bioassay | |
Corophium bioassay | |
OEB elutriate bioassay |
Water | |
---|---|
Determinand | Status |
Copper | |
Zinc | |
HCH | |
Dieldrin | |
Cypermethrin | |
PCB (passive sampling)? | |
PAH (passive sampling)? | |
Oyster embryo bioassay | |
Sea urchin embryo bioassay |
Gastropods | |
Determinand | Status |
TBT | |
Imposex/intersex (VDSI/ISI) |
Mussels | |
---|---|
Determinand | Status |
Cadmium | |
Mercury | |
Lead | |
Copper | |
Zinc | |
PCB | |
PAH | |
BFR | |
Lysosomal stability | |
AChE | |
Micronucleus | |
Histopathology/ gametogenesis | |
Stress on stress |
Fish | |
---|---|
Determinand | Status |
Cadmium | |
Mercury | |
Lead | |
Copper | |
Zinc | |
PCB | |
BFR | |
PAH metabolites | |
EROD | |
VTG | |
AChE | |
Liver histopathology | |
Macroscopic liver neoplasms | |
Intersex | |
External fish disease |
Step 2 : Integration of determinands by matrix for a given site
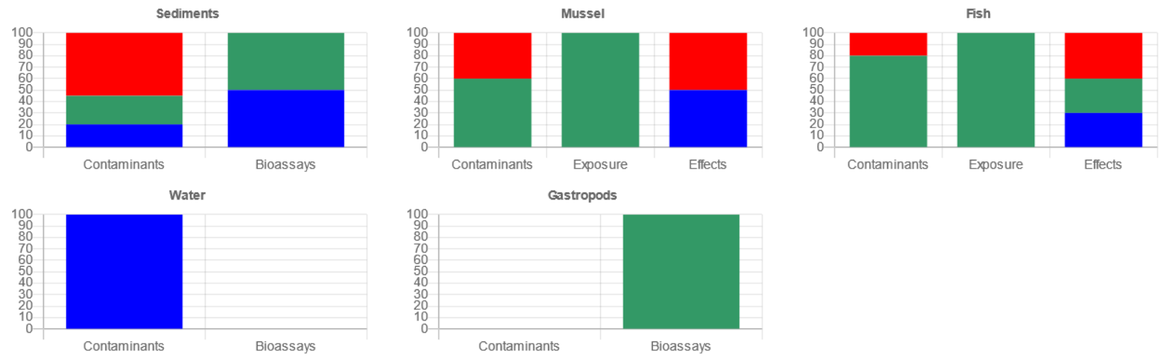
Step 3 : Integration of matrices for site assessment
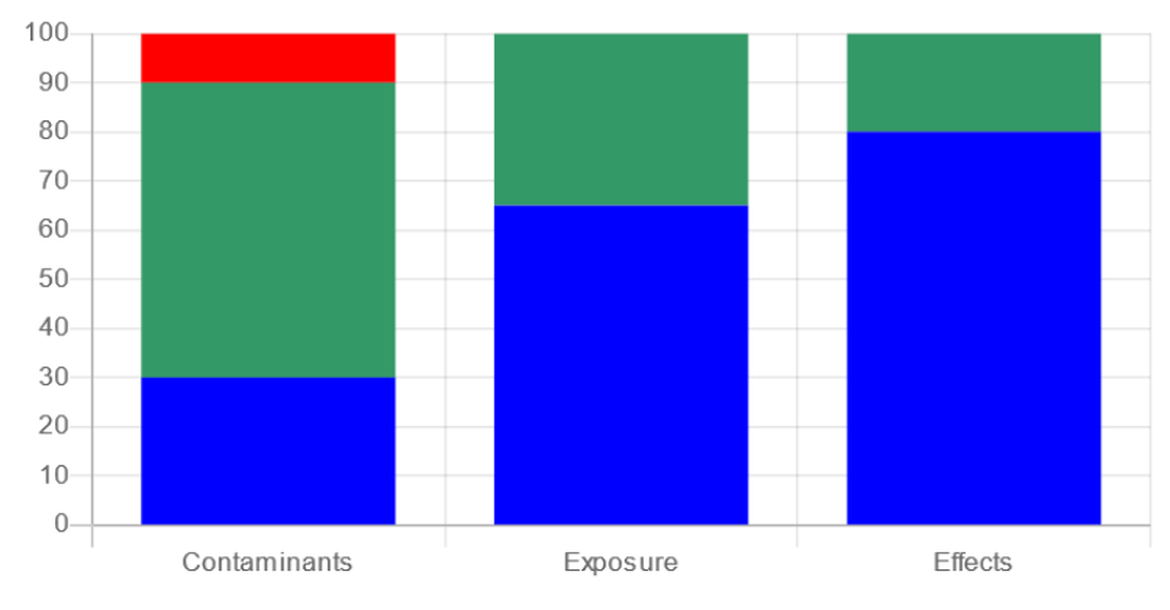
Step 4 : Overall assessment for site assessment
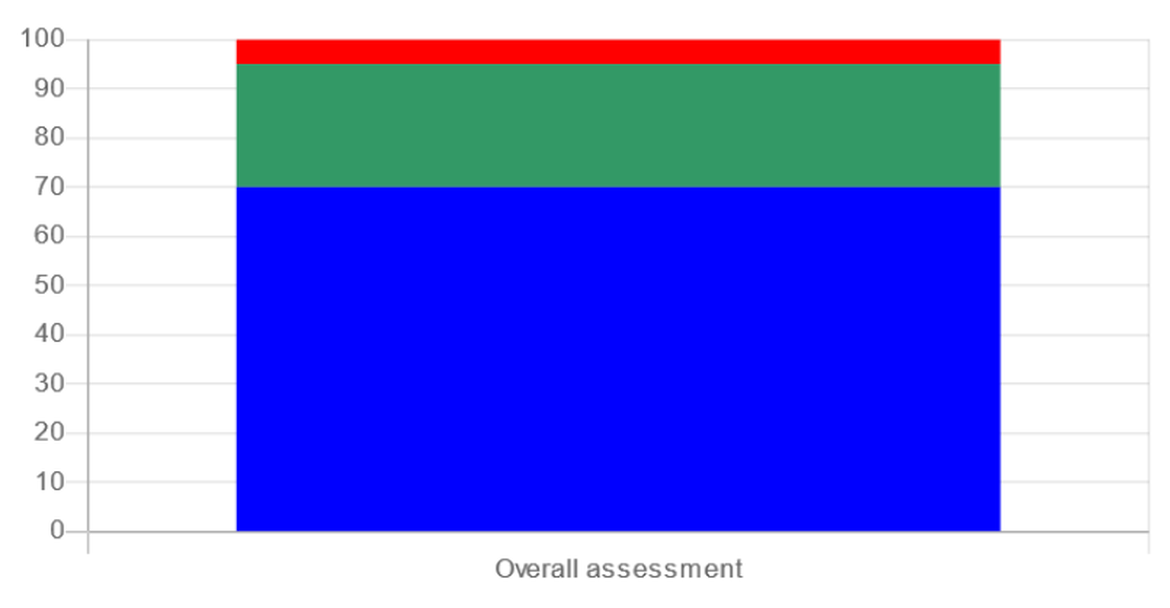
Methodology of the integrated assessment framework according Step 1 to 4 (Davies & Vethaak 2012)
Table 1: Assessment criteria used for the integrated assessment
Chemical compounds: Naphtalene (Naph), Benzo[a]anthracene (BaA) ,Benzo([a]pyrene (BaP, Fluoranthene(Flt), Phenanthrene (Phe), Anthracene (An), Chrysene (Chr), Benzo(a)perylene (BaPyr), benzo[ghi]perylene (BghiP), Pyrene (Pyr), Indium phosphide (Inp), Tributyltin (TBT), Perfluorooctane sulfonate (PFOS), Polybrominated diphenyl ethers congeners (Sum BDE), Polychlorinated biphenyls congeners , Terephthalate (TPhT)(CB28,52,101,105,118,138,153,156,180), Hexachlorohexans α- HCH and γ-HCH, Dichlorodiphenyldichloroethylene (p,p’-DDE), Hexachlorobenzene (HCB), dibenzothiophene (DBT) Mercury (Hg), lead (Pb), cadmium (Cd), arsenic (As), copper(Cu), zinc (Zn),
Biomarkers and bioassays: Metabolite polycyclic aromatic hydrocarbon 1-0H pyrene(PAH 10Hpyr), Metabolite polycyclic aromatic hydrocarbon 1-0H phenanthrene (PAH 10HPhe), Sex imposition and vas deferens (Imposex), World Health Organization -Toxic equivalents (Who-TEQ), Ethoxyresorufin-O-deethylase activity (EROD), cytochrome P450 IA (CYP1A), delta-aminolevulinic acid dehydratase (ALA-D), Glutathione reductase (GR), micronucleus frequency (MN), DNA strand breaks (comet assay), acethylcholinesterase activity (AChE), lysosomal membrane stability (LMS) and histological hepatic lesions, Fish Disease Index (FDI), Stress on Stress (SoS), Scope for growth (SFG), and Metallothionein (MT).
Table 2 corresponds to Step 1. It illustrates particular chemical contaminants and biological effects which exceed EAC and are red. Table 2 shows a diversity in the selection of species and a diversity in the selection of chemical contaminants and biomarkers, bioassays depending on the country. Each country selects chemical contaminants, biomarkers and bioassays from a core list (Davies & Vethaak, 2012) and assessment criterias are used to interpret biological effect according to its own local strategy. Despite this diversity, the common integrated approach makes it possible to compare the biological effects of contaminants measured locally. The integration of this approach into the Coordinated Environmental Monitoring Programme (CEMP), therefore making it mandatory for OSPAR countries to monitor biological effect, would greatly optimise the acquisition of new biological data and allow to optimise the comparison of stations over a wide geographical scale.
Figure 1 shows the Step 4 and illustrates the potential for applying such an integrated approach in eight countries. At each station, different species and matrices were selected among which: seven fish species (Gadus morhua, Limanda limanda, Melanogrammus aeglefinus, Plathychtys flesus, Pollachius virens, Solea solea, Zoarces viviparous), 2 mussels (Mytilus edulis, Mytilus galloprovincialis and the undetermined Mytilus sp.), 3 gastropods (Buccinum undatum, Nucella lapilus, Hinia reticulata), and sediment and water bioassays (Table 3). The interpretation is carried out according to the variability of the percentages characterising the chemical exposure and the biological effects on a North-South scale from Iceland to the North-Western Mediterranean. The most impacted stations in Figure 2 are the ones with the highest percentage of the determinants (biomarkers, bioassays and chemical contaminants) in red values, especially the ones exceeding 40% (up to 67%) parameters EAC or 3 times higher than BAC (four Danish stations: Randers fjord, Frederisksvaerk Roskilde Fjord, Kavelod, Langerak and one French station: Seine Bay) and to a slightly lesser extent the one between 30 and 40% of parameter in red (one Danish station: Roskilde Fjord, one Icelandic station: Reykjavik harbour, one French station: Loire Bay and one Scottish station: Firth of Forth). The assessment procedure shows that the chemical concentration was not always correlated with biological effects. This could be explained by the fact that only a few percentages of contaminants are measured on all contaminants present in the marine environment. This lack of clear correlation between biological response and chemical contamination could also be due to the delay in biological responses, since biological responses can be induced by exposure to toxicants at an earlier life stage. This does not constitute a handicap for monitoring because it is the overall chemical pressure which is assessed. The chemical contaminants measured nevertheless provide information on the overall chemical pressure. Some contaminants such as CB118, cadmium, lead or mercury appear most often in red and can be interpreted in relation to biological effects also in red such as neurotoxicity, genotoxicity or cytotoxicity. The imposex appears in red on the Roskilde and Langerak stations in Denmark and was in line with high TBT concentrations.
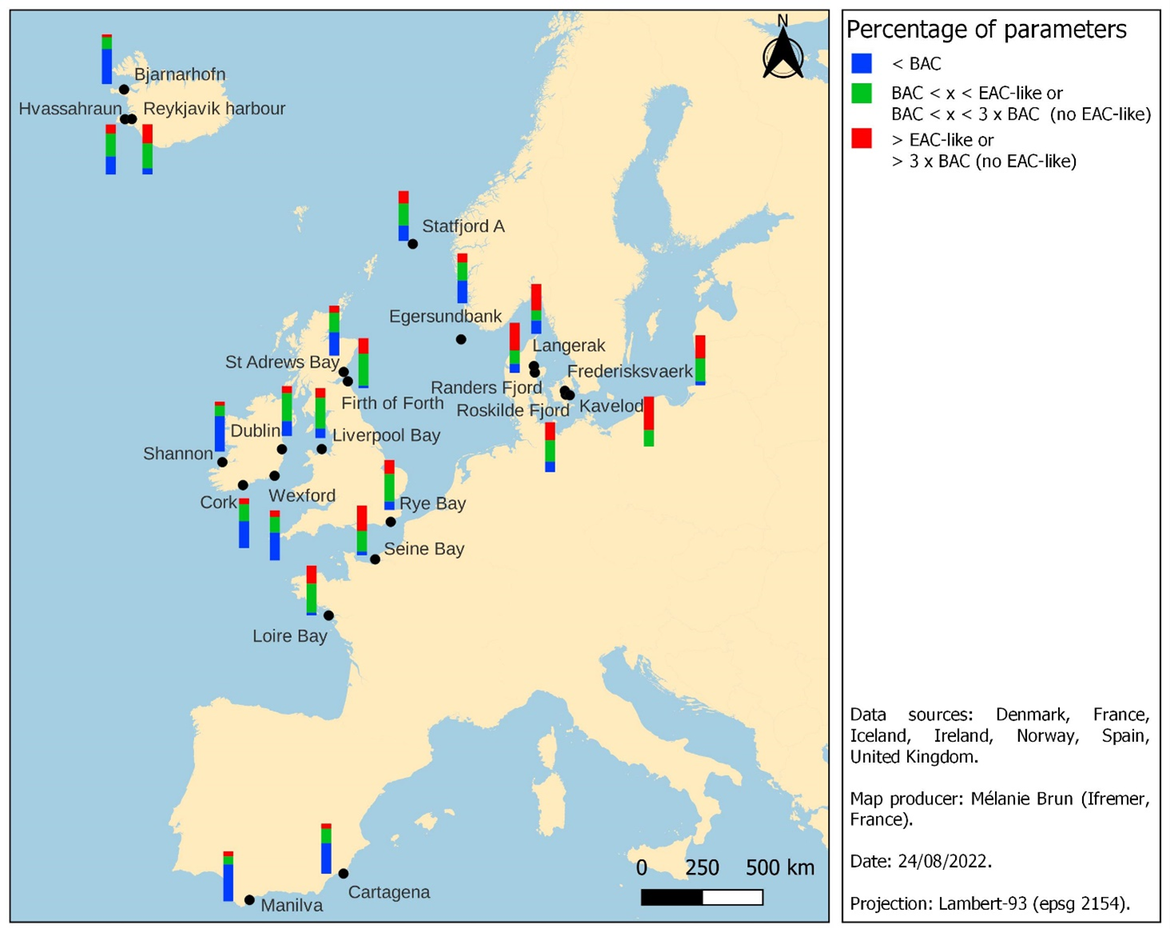
Figure 1: Integrated assessment in 22 stations from the North-East Atlantic and Mediterranean seas (data collected between 2010 and 2019)
Figure 2: Integrated assessment for each of the twenty-two stations
Matrices - Sediment (1), Limanda limanda (2), Plathychtys flesus (3), Solea solea (4), Zoarces viviparus (5), Clupea Harengus (6), Pollachius virens (7), Merlangius Merlangus (8), Gadus morhua (9), Nucella lapilus (10), Melanogrammus aeglefinus (11), Mytilus edulis (12), Mytilus galloprovinalis (13), Mytilus sp (14) and Water (15).
Data were collected between 2010 and 2019 in the OSPAR area (and extended to the Mediterranean sea to broaden and harmonize our expertise with the Barcelona Convention). The histograms represent the chemical contaminants and the biological effects measured on water and sediment with bioassays on amphipod, polychaeta, copepod, oyster, mussels, marine urchin, marine algal growth and on biomarkers with eight species of fish, two species of mussels and a gastropod. (As indicated in Table 1, not all the same species are measured at each station. A species core is primarily measured fish, mussels and gasteropod). Colour code was developed by Davies & Vethaak (2012).
Table 3: Species and matrices monitored in 8 European countries and 22 stations
Species/Matrix | Country | Station | |
---|---|---|---|
Fish | |||
Gadus morhua | ![]() | Norway | Statfjord A, Egersundbank |
Limanda limanda | ![]() | France | Seine Bay |
Ireland | Wexford; Dublin; Shannon; Cork | ||
UK | Firth of Forth; St Andrews Bay; Liverpool Bay; Rye Bay | ||
Melanogrammus Aeglefinus | ![]() | Norway | Egersundbank |
Platchthys flesus | ![]() | France | Seine Bay |
UK | Firth of Forth; St Andrews Bay | ||
Pollchius virens | ![]() | Norway | Egersundbank |
Solea solea | France | Seine Bay, Loire Bay | |
Zoarces viviparous | ![]() | Denmark | Randers Fjord; Roskilde Fjord; Frederisksvaerk Roskilde Fjord; Kavelod; Langerak |
Bivalves | |||
Mytilus sp. | ![]() | Denmark | Roskilde Fjord; Frederisksvaerk Roskilde Fjord; Langerak |
France | Seine Bay, Loire Bay | ||
Iceland | Bjarnarhofn; Hvassahraun; Reykjavik harbour | ||
Ireland | Wexford; Dublin; Shannon; Cork | ||
Norway | Statfjord A | ||
Spain | Cartagena; Manilva | ||
UK | Firth of Forth; St Andrews Bay | ||
Gasteropods | |||
Buccinum undatum | ![]() | Denmark | Randers Fjord |
Hinia reticulata | ![]() | Denmark | Langerak |
Nucella lapilus | ![]() | Denmark | Roskilde Fjord |
France | Seine Bay, Loire Bay | ||
Ireland | Wexford; Dublin; Shannon; Cork | ||
Matrix | |||
Sediment | France | Seine Bay, Loire Bay | |
Iceland | Reykjavik harbour | ||
Ireland | Wexford; Dublin; Shannon; Cork | ||
UK | Firth of Forth; St Andrews Bay; Liverpool Bay; Rye Bay | ||
Water | Ireland | Shannon; Cork |
We have chosen to illustrate the final result of the four steps (Step 4) of integration for the sake of simplification at the risk of frustrating an overly integrated result. However, this integrated approach can be used to trace the source of each chemical contaminant and biological effect measured on each species and sediment at the same station (Table 2). The methodology described by Davies & Vethaak (2012) makes it possible to reverse each step from Step 4 to Step 1 of integration to specify on each station and each species or sediment, the specific contribution of each chemical contaminant and each biological effect (mechanism of toxicity).
Guidelines should be regularly reviewed with innovative tools and updated analytical methods, assessment criteria. Guidelines should also recommend a minimum number of species, an appropriate geographical coverage (e.g. minimal sampling sites per region) and frequency, as well as appropriate statistical approach at every integration step from the local assessment to the wider scale regional assessment (Hylland et al., 2017a). Regular revision of the integrated monitoring guidelines give the opportunity to communicate with policy makers on how to meet their expectations and requirements for hazardous substances effect monitoring and assessment in the framework of the OSPAR Convention and the Marine Strategy Framework Directive).
3. Chemical stress scenarios in coastal areas
3.1 Case study in the Bay of Seine (France): A different stress adaptation effort depending on the sentinel species in the same habitat
Since 2017, France has developed and implemented the monitoring programme SELI (French Monitoring of Biological Effects Induced by Chemical Contamination) which is dedicated to the assessment of biological effects of chemical contaminants in flatfish and mussels. SELI was designed based on the ICON project (Burgeot et al., 2017), a demonstration of the chemical-biological integrated approach on a large geographical scale in the North-East Atlantic initiative (2008-2009). SELI completes the imposex mandatory monitoring undergoing since 2003 and the sediment and bivalve chemical monitoring (ROCCH) deployed under the CEMP. SELI also addresses descriptor 8 criteria 2 requirement (effects of contaminants) of the MSFD. For the SELI survey, flatfishes and mussels are sampled at stations close to estuaries likely to bring contrasted pressures in the Atlantic. A core set of biomarkers targeting early effects on main biological functions were measured: reprotoxicity (intersex), genotoxicity (micronucleus frequency and DNA strand breaks -comet assay-), neurotoxicity (AChE inhibition), cytotoxicity (lysosomal membrane stability) and histological hepatic lesions. This list was completed with a specific biomarker of exposure (PAH metabolites in fish bile), and with chemical contaminants concentrations.
In France, 50% of the traffic, 26% of the population, 40% of the economic activity and 30% of the agricultural activity is concentrated in the Seine catchment area (Dauvin et al., 2007). With autumn and winter floods exceeding 2 200 m3. s-1, anthropogenic river pressure is based on the discharges of two large urban agglomerations - Paris and Rouen - as well as the largest French seaport of the Atlantic - Le Havre. In this context of high anthropogenic pressure, the organisms are subjected to numerous sources of stress throughout their life cycle. The first SELI sampling cruises in the Bay of Seine (one of the two SELI sites) was conducted in 2018 (Mauffret et al., 2021; https://archimer.ifremer.fr/doc/00688/80024/) ).
The integrated approach developed in Davies and Vethaak (2012) was adapted to the French data, including temporal trends using the historical bivalve survey (annual survey since 1979, data used for temporal trend analysis from 2000), and the biological effects in gastropods, bivalves and fish (Figure 3). In the Seine estuary, in 2018, SELI survey with mussels and 2-3 years old flatfishes showed several significant early signs of chemical toxicity at sub-individual level as genotoxicity (DNA strand breaks), neurotoxicity (AChE inhibition) and cytotoxicity (LMS) biomarkers exceeded their thresholds. The integrated approach showed a decrease in chemical concentration biomarker alterations (biological effects) with distance from the Seine River mouth (Figure 3). However, organisms carry an individual bioaccumulated chemical load and associated physiological stress beyond the river mouth.
Different species’ abilities to cope with stress were observed. Sole (Solea solea, Soleidae) were the most (commonly) trawled flatfish in the studied area, as compared to two Pleuronectidae species also found in the area, flounder (Platichthys flesus) and dab (Limanda limanda). Sole showed lower body burdens of most chemicals (Hg, Cd, Zn, PCB, chlorinated pesticides, PBDE and HBCDD) than flounder and dab. However, concentrations of three chemicals (Ag, Cu and perfluorinated compounds) were higher in sole than in flounder and dab. Concentration of the hydroxypyrene metabolite was higher in flounder than in dab and sole. These differences in chemical impregnation were possibly due to different metabolic capabilities between flatfish families (Soleidae and Pleuronectidae) and/or different habitats and different life cycle characteristics, e.g., the flounder is more estuarine than the two other species. Although sole had the lowest chemical concentrations for several chemical families, they showed the most altered responses regarding genotoxicity (DNA strand break) and neurotoxicity (AChE enzymatic activity, together with the dab). S. solea is a sensitive and widely spread species in the studied areas, therefore likely to be a suitable candidate as sentinel species in France. However, the use of sole as sentinel species requires the development of specific BAC and EAC. These original findings on the health status of the sole, flounder and dab illustrate the diversity of biological effects induced by chemical mixture.
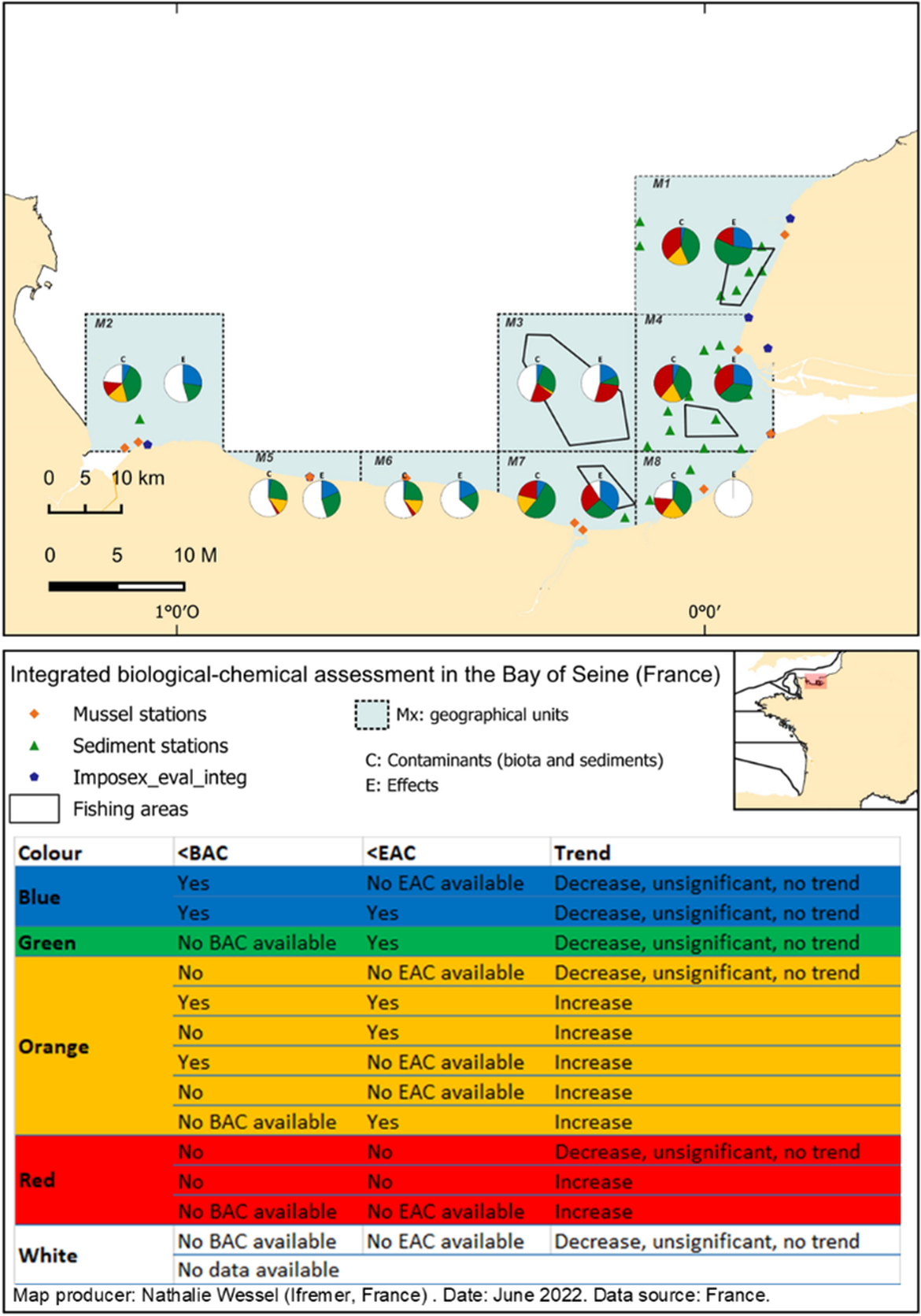
Figure 3 :Integrated biological-chemical assessment in 8 geographical units from the Bay of Seine (France) including contamination (C) of sediment and biota (bivalves and fish) as well as biological effects (E) in fish, mussels and gastropods with traffic light determination according to the table below the map.
C: Contamination was assessed by comparing contaminant levels in sediments, bivalves and fish with available thresholds (BAC or EAC-like) and by considering temporal trends in bivalves (annual data since 2000 were used). Contaminants (C) include PAH (8 OSPAR common indicator PAH), trace elements (7: Cd, Cr, Cu, Hg, Ni, Pb and Zn), PCB (7 OSPAR common indicators and 2 additional with BAC values), brominated compounds (6 OSPAR common indicator PBDE and HBCDD), pesticides (DDEpp’ and lindane), PFOS and TBT. E: Effects were assessed by comparing levels of biomarkers in fish, mussels and gastropods. Effects (E) include AChE, DNA strand break, Lysosomal Membrane Stability, micronucleus, PAH metabolites and imposex. Not all determinants are analyzed in each matrix; the C pies integrate 85 contaminant*matrix combination and the E pies integrate 11 biomarker*species combination.
3.2 Integrated Assessment of the Firth of Forth and St Andrews Bay, Scotland: Fish pathologies and genotoxicity in mussels.
The Firth of Forth is located on the east coast of Scotland and runs into the North Sea. It is a highly industrialised area with historically high levels of contaminants including PAHs and heavy metals, especially mercury. The main source of contaminants in the marine environment there include inputs of domestic, industrial and refinery effluents but also runoff and atmospheric deposition.
In this integrated assessment, concentrations of contaminants and their biological effects were measured in sediment, fish and mussels. Biomarkers of combined different biological effects were analysed and included: PAH metabolites in fish indicating recent exposure to PAHs; two indicators of genotoxicity – assessment of micronuclei in fish and the comet assay in mussels which measures DNA damage; the fish disease index (FDI), an indicator of the general health of the fish population; and two general stress biomarkers in mussels, stress on stress (SoS, i.e., mussel survival time in the air) at a whole organism level and lysosomal membrane stability at a cellular level. Contaminant analysis included metals, PAHs, PCBs and PBDEs. Data from a single time point for each determinant between 2017 and 2020 were compared to BAC and/or EAC. Results from the Firth of Forth were compared to a nearby control site, St Andrews Bay (Figure 4).
The majority (47/63) of contributing analyses to the Firth of Forth integrated assessment gave acceptable results (blue/green). Two biomarkers gave unacceptable results (red, >EAC), FDI in dab Limanda limanda and one of the genotoxicity indicators (mussels). The contaminant analyses indicated that concentrations were elevated above acceptable levels (red, >EAC) in 14 of the analyses across a wide range of contaminants including some metals, PCBs and PAHs. The St Andrews Bay control site had fewer (7) red results including some metals and PCB concentrations and one biomarker, the genotoxicity indicator in mussels.
The integrated assessment of the Firth of Forth (Figure 4) showed that although most of contaminants and their biological effects are at levels which are not causing significant harm, some contaminants are at unacceptable concentrations (red, >EAC). Compared to St Andrews Bay fewer determinants were at background (blue, <BAC) and more were elevated above background at either acceptable (green, >BAC) or unacceptable (red, >EAC) levels. This can be expected given the higher level of industry currently present in the Forth and historically. The integrated assessment approach is a useful way to demonstrate this, combining biological effects of a diffuse chemical contamination and representing data across multiple sites and from a wide range of matrices. This integrated approach also makes it possible to guide targeted vigilance on the frequency of fish population pathologies and specific genotoxic effects on mussels.
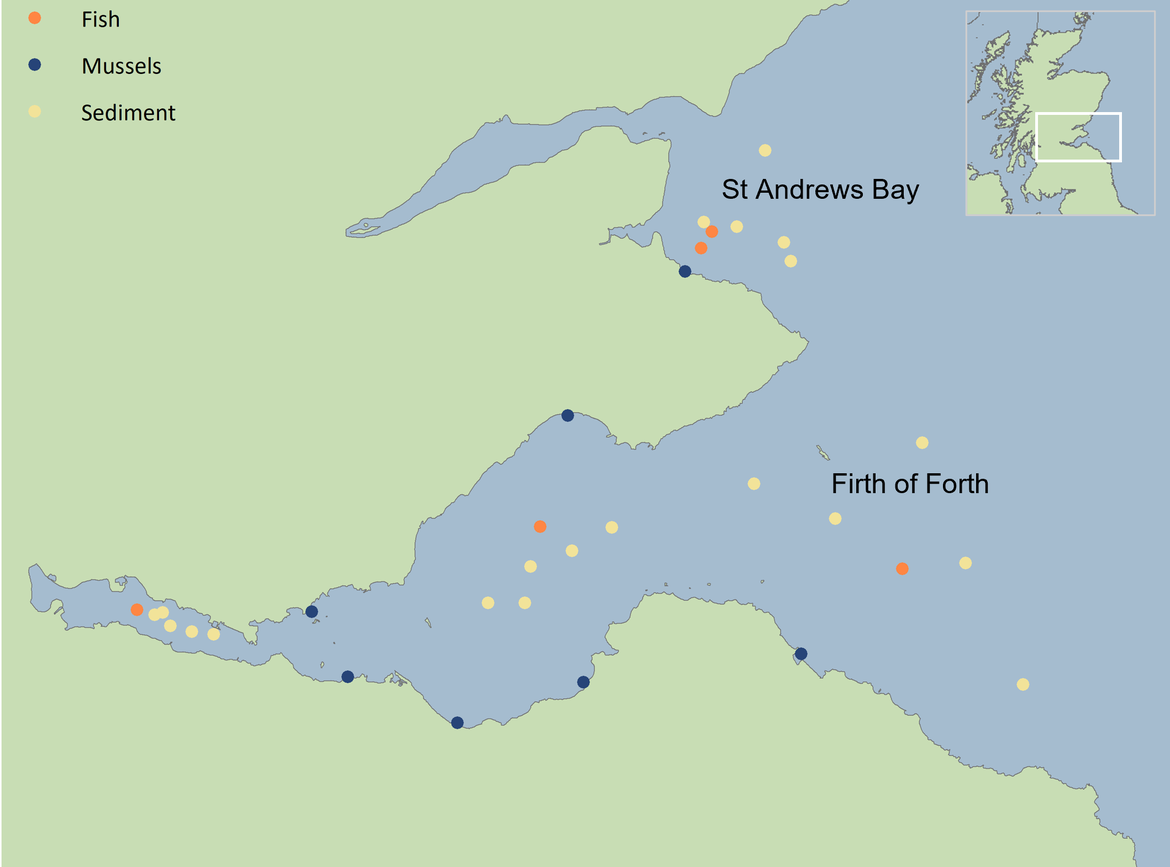
Figure 4: Integrated assessment of the Firth of Forth and St Andrews Bay (Scotland, UK). Overall assessment histograms for each sampling area show the proportion of analyses (n=63) that were at background (blue, <BAC) or elevated above background at either acceptable (green, >BAC) or unacceptable (red, >EAC) levels. Sites included in each sampling area are shown (circle).
3.3 Tributyltin: The story of a hidden bottom-up killer of the Crangon crangon population in the Southern North Sea
The application of the extremely toxic biocide tributyltin (TBT) as an antifouling agent (Figure 5) has caused, since the end of the 1970s, visible fertility and calcification impairments in oysters and induction of imposex in female gastropod snails, i.e. females develop a penis and become infertile, leading to the decline and local extinction of sensitive mollusc species. These biological effects of TBT observed in the field provided strong evidence on the occurrence of endocrine disruption in the aquatic environment and resulted in an international ban on TBT in 2008 signed by signatory countries of the IMO Convention (ca. 96% of the gross tonnage of the world’s merchant fleet). This case study is particularly interesting as it is one of the few examples demonstrating the specific biological effects of a chemical contaminant. For these same reasons, it also differs from the other case studies presented in this chapter as it is specific to one chemical (TBT) and concern one biomarker (imposex), while the other cases presented are based on multi-biomarkers approach with the intention to track the effect of the chemical pressure as a whole.
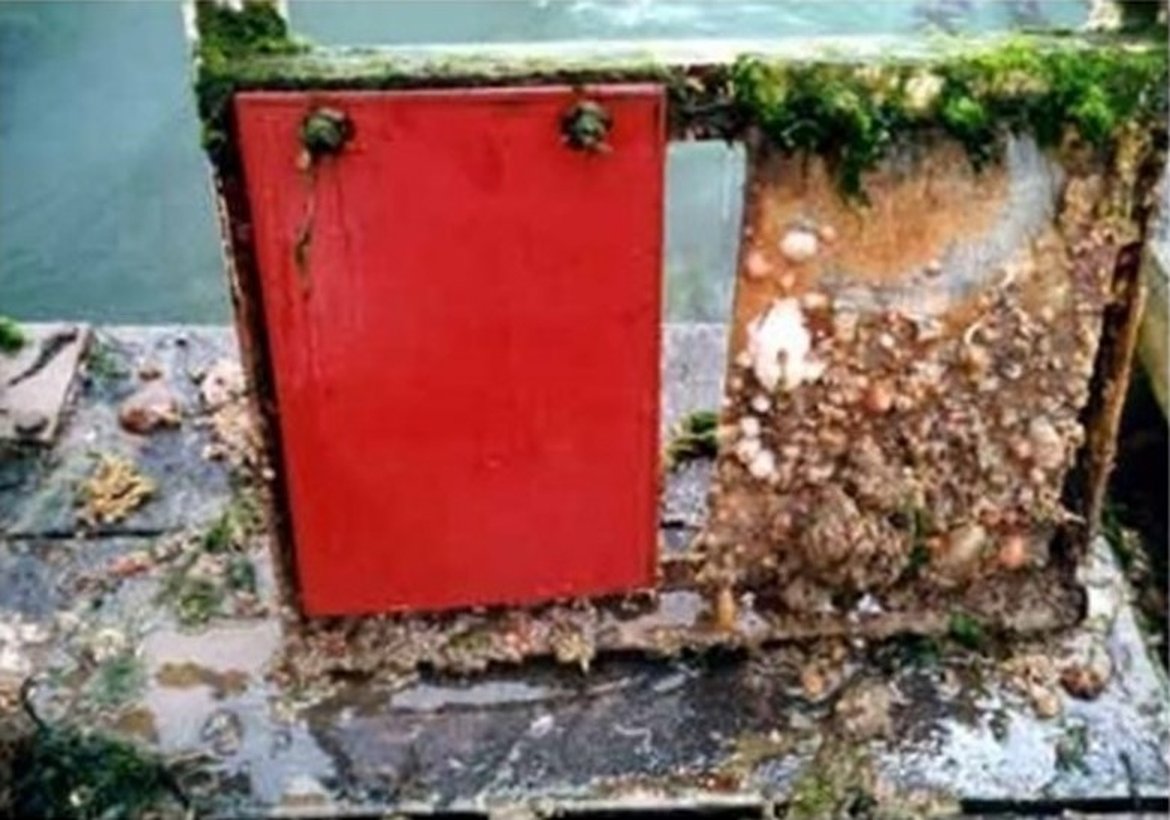
Figure 5: Evidence of the antifouling effect of tributyltin (TBT): absence of biofouling on the left panel, painted with TBT-based antifouling; accentuated biofouling on an unpainted panel, on the right.
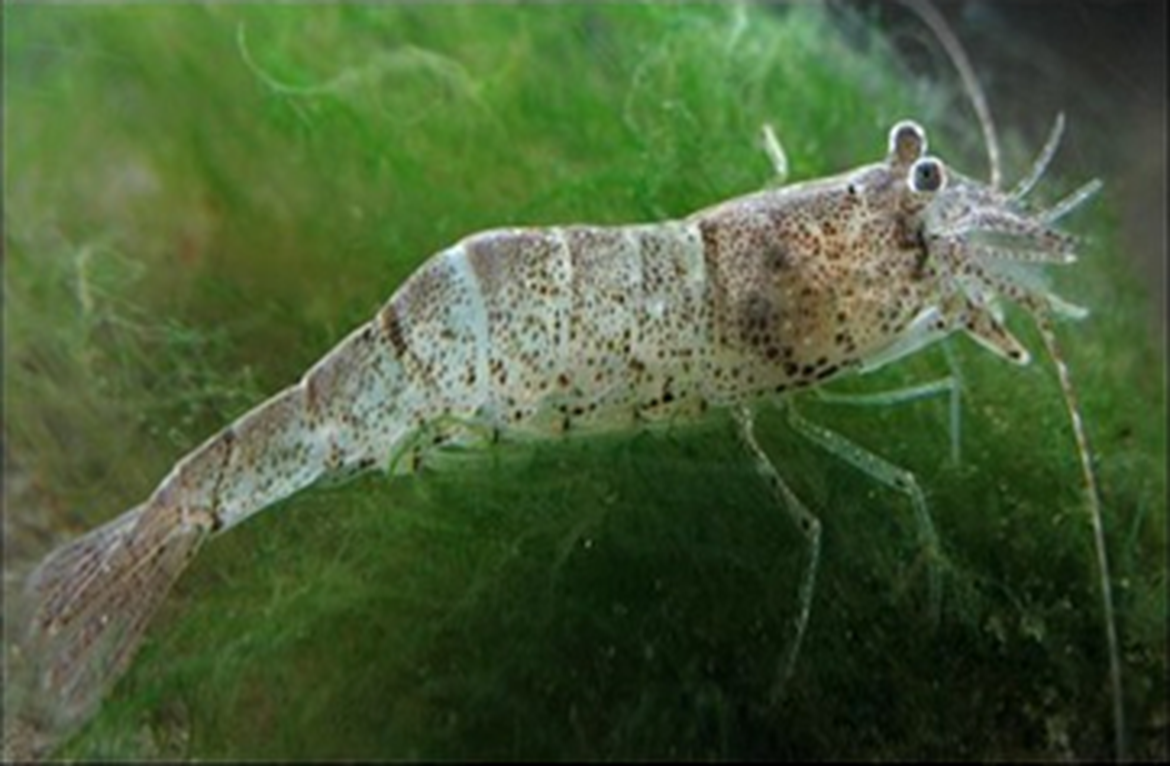
Figure 6: European shrimp Crangon crangon (Linnaeus, 1758)
Recently, it has been reported that TBT also affects crustacea such as C. crangon’s population, which is mainly distributed in European waters, including the Black Sea and considered as one population at genetic level. A recent retrospective data analysis, obtained over 60 years with the crustacean Crangon crangon's (Figure 6) life history in the Southern North Sea (Luttikhuizen et al., 2008 and ICES report 2014, Parmentier et al., 2020), pointed to a formerly unnoticed large geographical scale TBT-induced endocrine disruption as the dominant population driver, more important than mortality and recruitment (Figure 7). An important driver of the widespread impairment are the metabolic interactions of TBT in the mollusc and shrimp taxa manifested by physical, functional and gene-expression blocking of the growth and reproduction processes in lower organisms. These metabolic impairments were shown to be extremely strong in the ovaries of C. crangon and formed the basis of the morphological impairments monitored by biomarkers. The current scientific knowledge argues that the metal-type fixation of ionized TBT in acidic intracellular pH conditions is the predominant process for its bioaccumulation, which could reach high body burdens and lead to severe impacts on the physiology of C. crangon (Parmentier et al., 2020).
TBT concentrations in well-aerated sediments have strongly decreased since the ban in 2008. However, the question of whether TBT prevalence is an important indicator of C. crangon‘s population health status, which has long been regarded as a non-endangered species, remains a topical issue today. Nowadays, overfishing and the landings of previously discarded undersized shrimp have been identified as the new threats since approximately 2014. The TBT story is a reference case study of the importance and necessity of long-term monitoring of ecosystem influences and the need for pragmatic integrations of unrelated drivers (Parmentier et al., 2020).
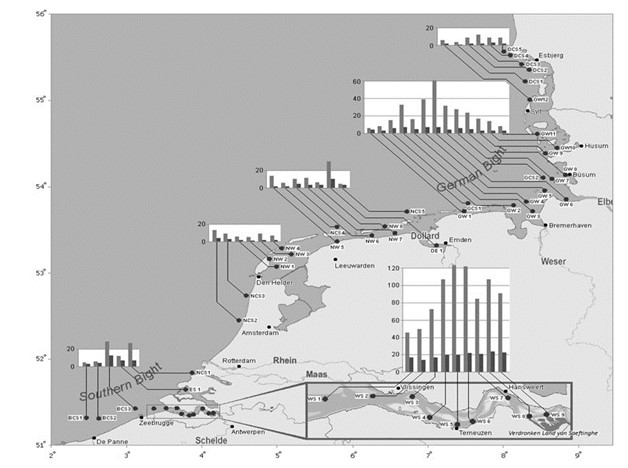
Figure 7: Spatial distribution of TBT (light gray bars) and triphenyltin (dark gray bars) concentrations in C. crangon muscle (Y-axis, in µg/kg dw) along the south coast of the North Sea in 2009 (Verhaegen, 2012; Verhaegen et al., 2012). The X and Y axes represent the longitude Northern length (NL) and the latitude Eastern length (EL), respectively.
4. Long-term series to confirm the biological effects of chemical contaminants in coastal and offshore areas
4.1 Why measuring biological effects on a pristine area in Sweden?
The site Fjällbacka is situated on the west coast of Sweden (Figure 8), ca. 150 km north of the City of Gothenburg. Absence of industrial activity or waste dumping and low human population density have qualified this area as a national marine monitoring reference station. In the Fjällbacka area the sentinel fish species eelpout (Zoarces viviparus) has been used in biological effects and chemical monitoring for 30 years.
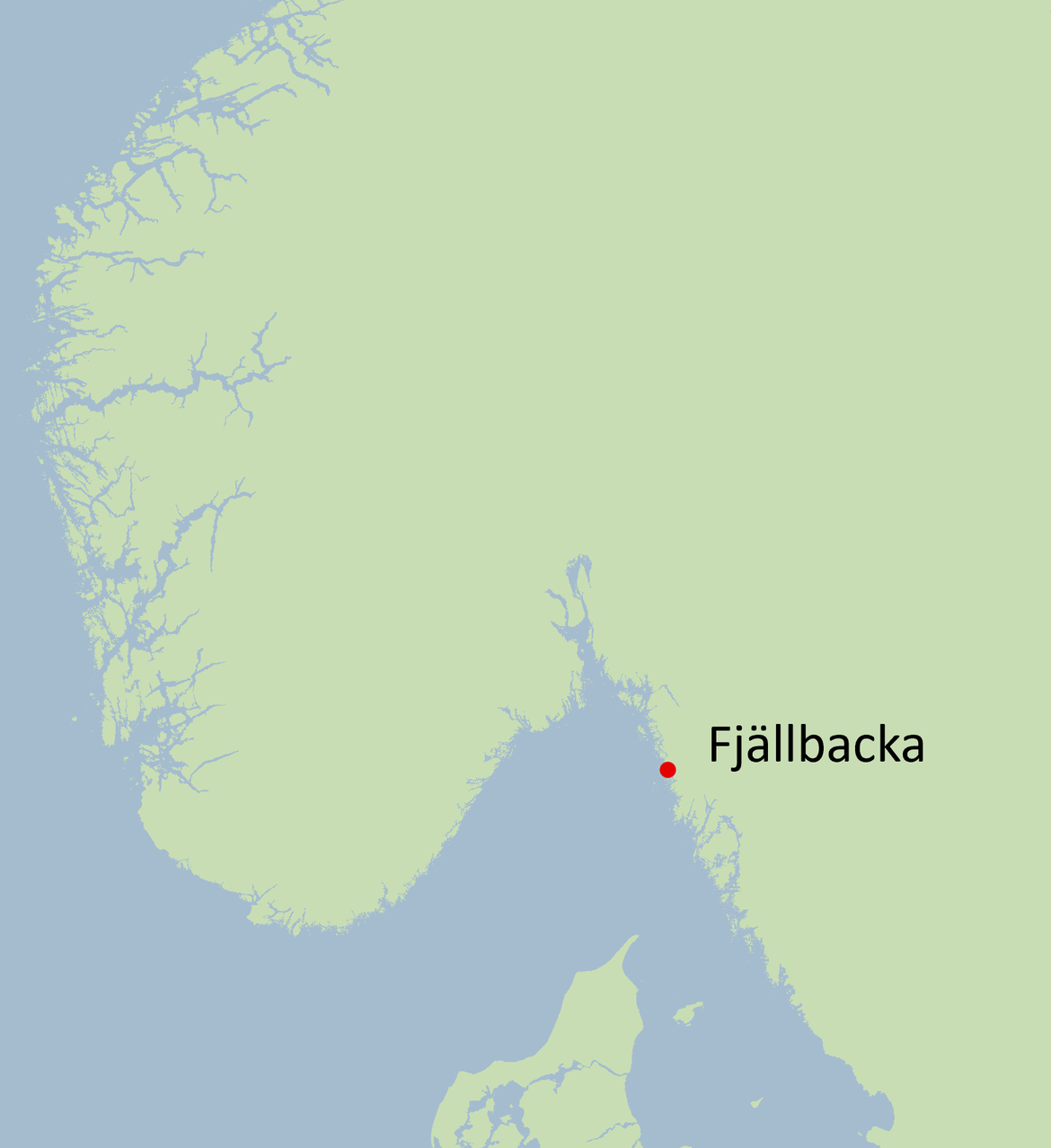
Figure 8: Fjällbacka sampling pristine site of the sentinel fish species eelpout (Zoarces viviparus) in Sweden
This time series (Figure 9) shows that the health status of the eelpout has been negatively affected but it has not been possible to point out any specific contaminants. Over the last years it seems as if the negative health development has halted. In the current integrated assessment of data from Fjällbacka, biomarkers not complying with EACs (in red, Figure 9) are mainly related to biological exposure parameters EROD, catalase and glutathione reductase activities, whereas biomarkers complying with EACs (in green, Figure 9) seem to be driven by the effect parameters GSI, LSI and vitellogenin which characterise reproductive and physiology disturbance. The blue colour is the effect of the contribution of the contaminants. It is noticed that the figure of the integrated data for the period 2010-2019 of the ten years annual monitoring of eelpout seem to indicate relative stability with exception of year 2017. The absence of red 2017 seems to be due to both low EROD and catalase activities. During the periods 2013-2016 the larger red piles are caused by higher EROD metabolic activities. During the same time period the two other exposure parameters catalase and glutathione reductase successively increase. In 2018 and 2019 the red colour is back again, and it seems to be caused by an increase in EROD and catalase activities. GSI was stable most of the time but increased the last 2 years caused by a two-three weeks later sampling date of the fish. For LSI a small decrease was seen during the period 2010-2019. For vitellogenin in the female, a small but significant decrease is seen for the period 2010-2019. It can be concluded that this integrated assessment shows that the eelpout from Fjällbacka are exposed to a mixture of contaminants that potentially affect the health of the fish, but it is not possible to point out any specific contaminant. It is worrying that the effects occur in a reference area considered relatively unaffected by direct human activity. It is therefore important to find out whether it is unknown contaminants (emerging contaminants not already monitored) or known contaminants (already monitored in other contaminated sites) that are not monitored today that cause the observed effects in this supposed low exposed site of Fjällbacka.
Figure 9: Integration of monitoring data in the eelpout fish sentinel species during ten years from Fjällbacka. Overall assessment histograms for the sampling area show the proportion of analyses that were at background (blue, <BAC) or elevated above background at either acceptable (green, >BAC) or unacceptable (red, >EAC) levels. Red colour is mainly explained with metabolism (EROD) and oxidant (CAT and GR)) stress.
4.2 Biological effects in cod from the Oslofjord
The Norwegian coastal monitoring programme is performed annually to determine the health status of the coastal waters with respect to chemical concentrations in sediments, water and biota as well as biological effects assessment in cod (Gadus morhua) from selected locations. An area of particular interest is the Oslofjord (Figure 10), which receives runoff from the urbanised area of the capital Oslo, including industrial and sewage treatment discharges as well as high density of shipping and boating activities. The perceived decline of the cod population within the Oslo fjord has also been an issue of recent concern. Whether this decline can be explained by poor water quality and/ or chemical contamination is important to determine. Biological effects monitoring using wild caught cod from within the fjord has been performed for approximately the last 10 years. The biomarkers of metabolism measured include 1) polycyclic aromatic hydrocarbons (PAH) metabolites, 2) ethoxyresorufin-O-deethylase (EROD), 3) cytochrome P450 IA (CYP1A) and delta-aminolevulinic acid dehydratase (ALA-D). The biomarkers provide an indication of exposure to chemicals such as PAH (PAH met, EROD and CYP1A) and lead (ALA-D). The long time series data from 2010 to 2019 have been used within the integrated assessment framework for contaminants and biological effects, which compares the measured values with the BAC and/or EAC values to provide an indication as to whether good environmental status has been achieved (Figure 11). Chlorinated biphenyls (PCBs) were the parameters responsible for the red columns, indicating that certain PCBs were above the EAC values. Although regulated, these compounds are still present in the environment and absorbed by cod. PCBs are mostly attached to fatty tissues where they tend to accumulate. They are partially metabolised into aryl oxides, which are highly reactive intermediates on the body’s molecules and cells. The follow-up from 2010 to 2019 shows that despite some variations, this trend does not decline. The integrated approach with chemical and biological effects shows an overall trend varying annually between 25% and 60% with (red) values (Figure 11) that exceed the chemical and biological (EAC, BAC) thresholds. Since 2010, biomarkers have confirmed a chronic chemical impact on the metabolic adaptability of cod. Although the combination of various anthropogenic and climatic pressures cannot be excluded in the Oslofjord, the biological effects of long-term chemical exposure appear to be one of the main sources of the perceived decline of the cod population.
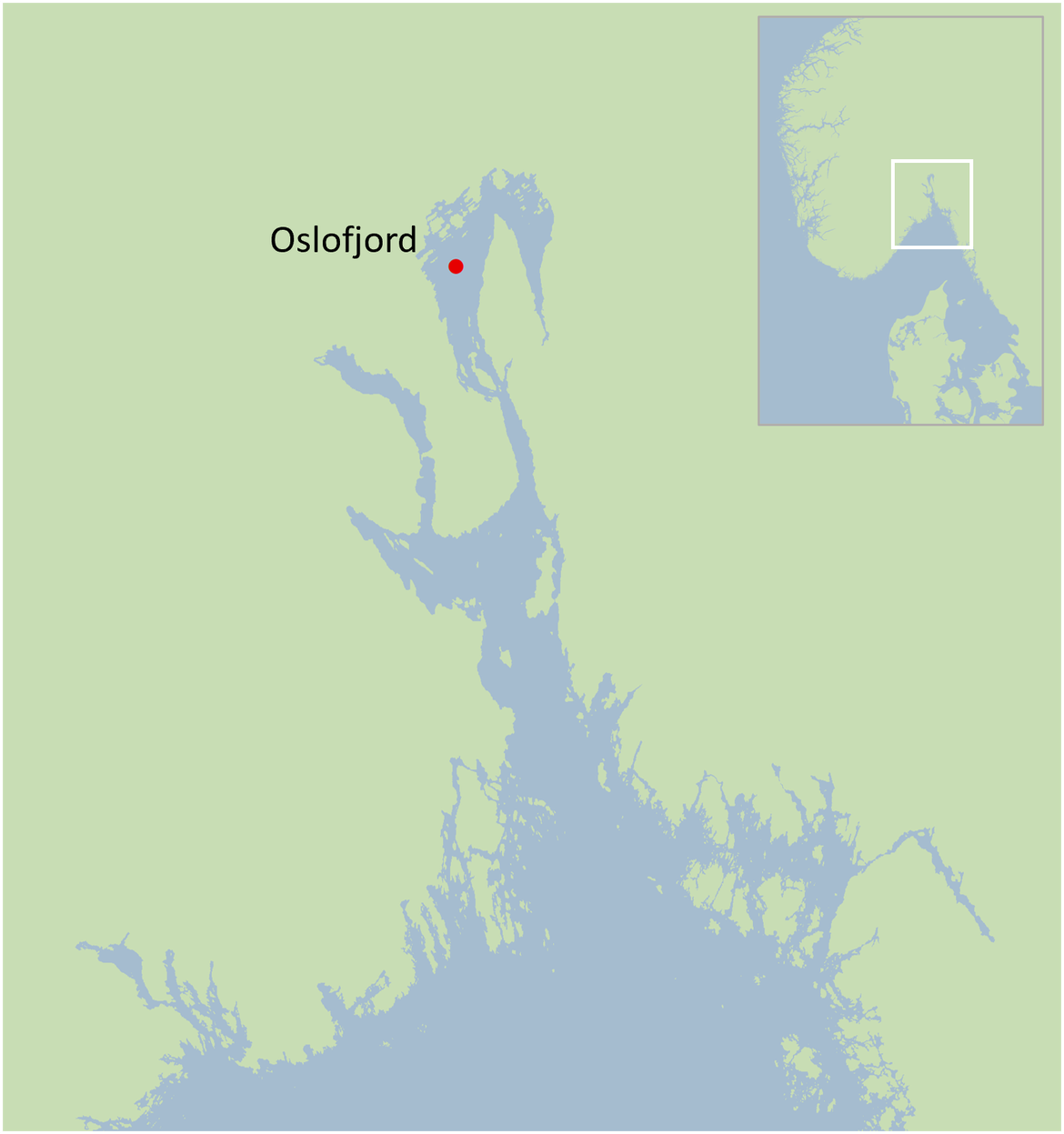
Figure 10: Cod sampling area in Oslofjord area in Norway
Figure 11: Integration of chemical concentrations and biological effects in cod from the Oslofjord between 2010 and 2019. Overall assessment histograms for the sampling area show the proportion of analyses that were at background (blue, <BAC) or elevated above background at either acceptable (green, >BAC) or unacceptable (red, >EAC) levels. Chlorinated biphenyls (PCBs) were the parameters responsible for the red columns with metabolic stress (EROD, PAH metabolites). Blue colour characterizes a normal metabolism and green colour shows a variation of the regulated metabolism.
4.3 Assessment of spatial and temporal data on diseases of the common dab (Limanda limanda) in the OSPAR Region using the fish disease index (FDI) approach
Systematic monitoring of diseases affecting fish species in the North Sea was very widely developed in the early 1980s. The quantification of external pathologies and macroscopic and microscopic liver lesions performed on benthic fishes such as the dab Limanda limanda and flounder Plathichtys flesus allowed to develop a Fish Disease Index original (Lang et al., 2017). Monitoring of fish diseases is now considered a useful component of programmes aiming to analyse and assess effects of environmental stressors, including hazardous substances, on ecosystem health. The original work carried out for more than thirty years in the North Sea on flounder and dab makes it possible today to apply a spatiotemporal indicator of long-term combined effects following the model of the Fish Disease Index.
For the analysis and assessment of disease data, the Fish Disease Index (FDI) approach was applied and the concept of BAC and EAC is used as detailed by Lang et al. (2017). In brief, the FDI summarises the disease status of an individual specimen by a single number that is calculated based on the presence/absence of a set of nine externally visible disease conditions commonly affecting dab, their intensity (expressed as severity grades) and their suspected impact on the host (by using disease-specific weighting factors generated through expert judgement) as well as adjusting factors compensating for effects of sex, length and sampling season on the disease prevalence. Based on the individual FDI values, mean FDI values are calculated for groups of fish. To assess the disease status of either an individual fish or groups of individual fish, the concept of Background Assessment Concentration (BAC) and Environmental Assessment Criteria EAC) is used as detailed by Lang et al. (2017).
The data clearly indicate marked differences between sampling stations both in terms of current health status and trends (Figure 12 and Figure 13). At some stations (N01, GB3, GB4, P01, P02, N04, N06, N10), current FDI values exceeded the EAC, reflecting a bad health status of dab associated with a loss in general fitness (decrease in condition factor). Some stations revealed an increasing trend in FDI (stations GB1, N01, GB3, GB4, P02, N04, B12), partly exceeding the EAC (stations N01, GB3, GB4, P02) which indicates a significant worsening of the health status over the past years (Figure 12). While some stations only relatively recently developed trends towards elevated FDI (N01 in Figure 13), others show long term trends for worse health status of dab (P02 in Figure 13). The latter station is located in proximity to the Ekofisk oil field at which elevated FDI in dab coincided with increased concentrations of mercury in muscle tissue of dab (Lang et al., 2017).
Monitoring of fish diseases is considered a useful component of programmes aiming to analyse and assess effects of environmental stressors, including hazardous substances, on ecosystem health. Through ICES/OSPAR activities, methods for fish disease monitoring are in place, quality assured through published guidelines and have been intercalibrated repeatedly. The FDI approach can be used to analyse and assess status and trends in health status of common dab from the OSPAR Maritime Area; it can also be applied to other fish species after modification, provided that data are available. Degradation of the health of the dab was identified by the FDI on specific areas. Multiple sources of pathology are difficult to pinpoint, but FDI is a global indicator of true ecological significance. It complements early biomarkers measured in cells and organs. Finally, although the pathologies are the result of a combined effect of multiple sources such as pathogens (viruses, bacteria, parasites) and various chemical contaminants, it is possible to identify a coincidence with high bioaccumulation of mercury in specific areas such as Ekofisk.
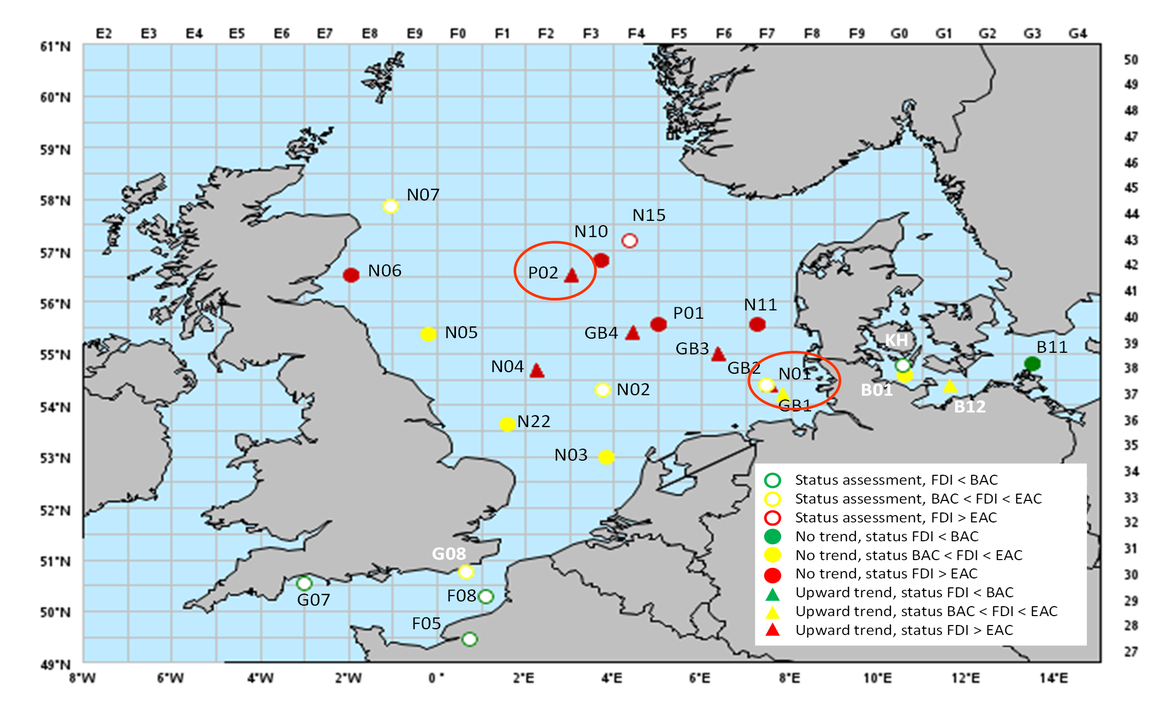
Figure 12: Results of the German fish disease monitoring programme 1998-2018: Status and trends in Fish Disease Index (FDI) for dab (L. limanda) from the North Sea and adjacent areas assessed by MIME.
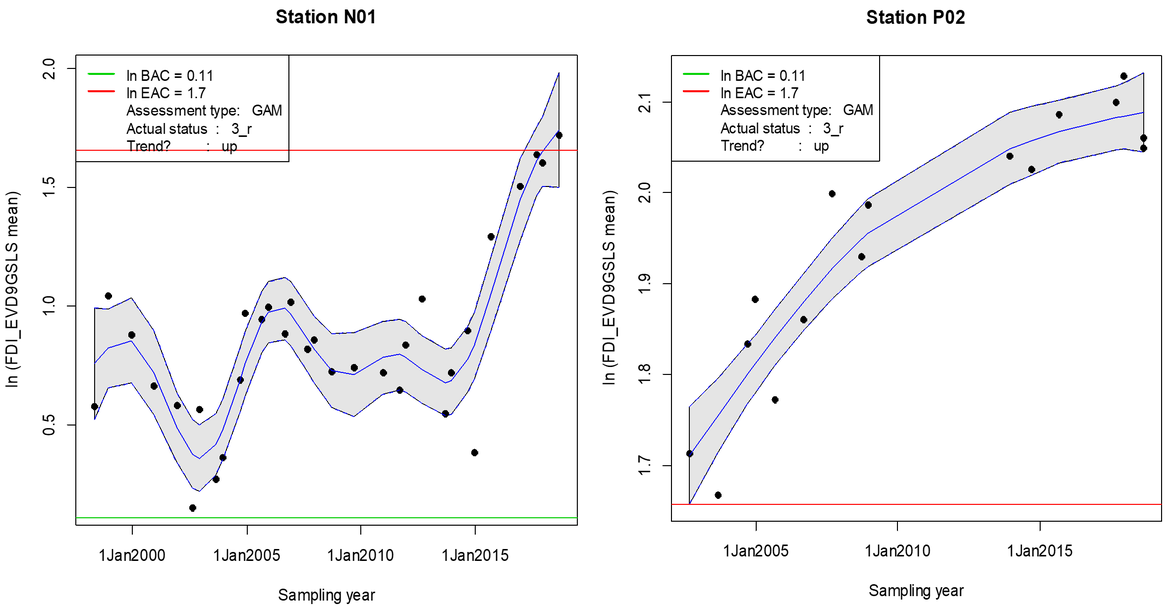
Figure 13: Status and trends in Fish Disease Index (FDI) for dab (L. limanda) at two stations (P02 and N01) over time (log-transformed mean values with confidence band, green horizontal line: BAC; red horizontal line: EAC) (for location of sampling station see Figure 12).
4.4 Development of methods to evaluate trends of liver neoplasms in the common dab (Limanda limanda) flatfish: a UK case study (2016-2020)
Several field and laboratory studies have previously demonstrated correlations between liver neoplasia (carcinogenesis) and exposure to hazardous chemicals (Hawkins et al., 1990; Myers et al., 1990; Myers et al., 1998; Stehr et al., 2004). The long-term monitoring of liver neoplasm prevalence in the common dab (Limanda limanda) flatfish has been conducted by several countries in the OSPAR Maritime Area including Germany, Scotland, England and Wales, and is regarded as a top-level indicator concerning the biological effects of contaminants. Whilst previous studies have shown correlations between hazardous chemicals and the formation of liver neoplasms, a robust approach for large scale geographical assessments of long-term monitoring data is yet to be developed. This has largely been determined by the issue concerning the positive influence of age on the formation of liver neoplasms i.e. the prevalence of liver neoplasms is higher in older fish (Myers et al., 1994; Stentiford et al., 2010). It is difficult to determine whether this is the result of increasing age or, since cancer is a chronic disease, whether it occurs because of a continued long-term chronic exposure to contaminants over time. Nevertheless, it is important that assessment tools consider the confounding effect of age so that any spatial and temporal differences are accurate and not indicative of age differences between those fish populations being assessed. For instance, in the UK, dab aged 3 to 6 years have been targeted in the monitoring of fish liver neoplasms (Figure 14).
There is a significant difference in liver neoplasms prevalence between UK biogeographic marine regions (Figure 14, Table 2). The application of rudimentary indicator response thresholds to the assessment period data (2010 to 2015), revealed the following:
- Prevalence of neoplasms at four out of five UK marine subregions have reduced by about 30% between the first period (2004-10) and the following assessment period (2011-2015).
- Irish Sea fish exhibit a ‘significant response’ (≥ 7,7% neoplasms in analysed population).
- Southern North Sea fish exhibit an ‘elevated response’ (3,88 to 7,74% neoplasms in analysed population).
- Eastern Channel, Northern North Sea, and the Western Channel and Celtic Seas fish exhibit a ‘background response’ (≤ 3,87% neoplasms in analysed population).
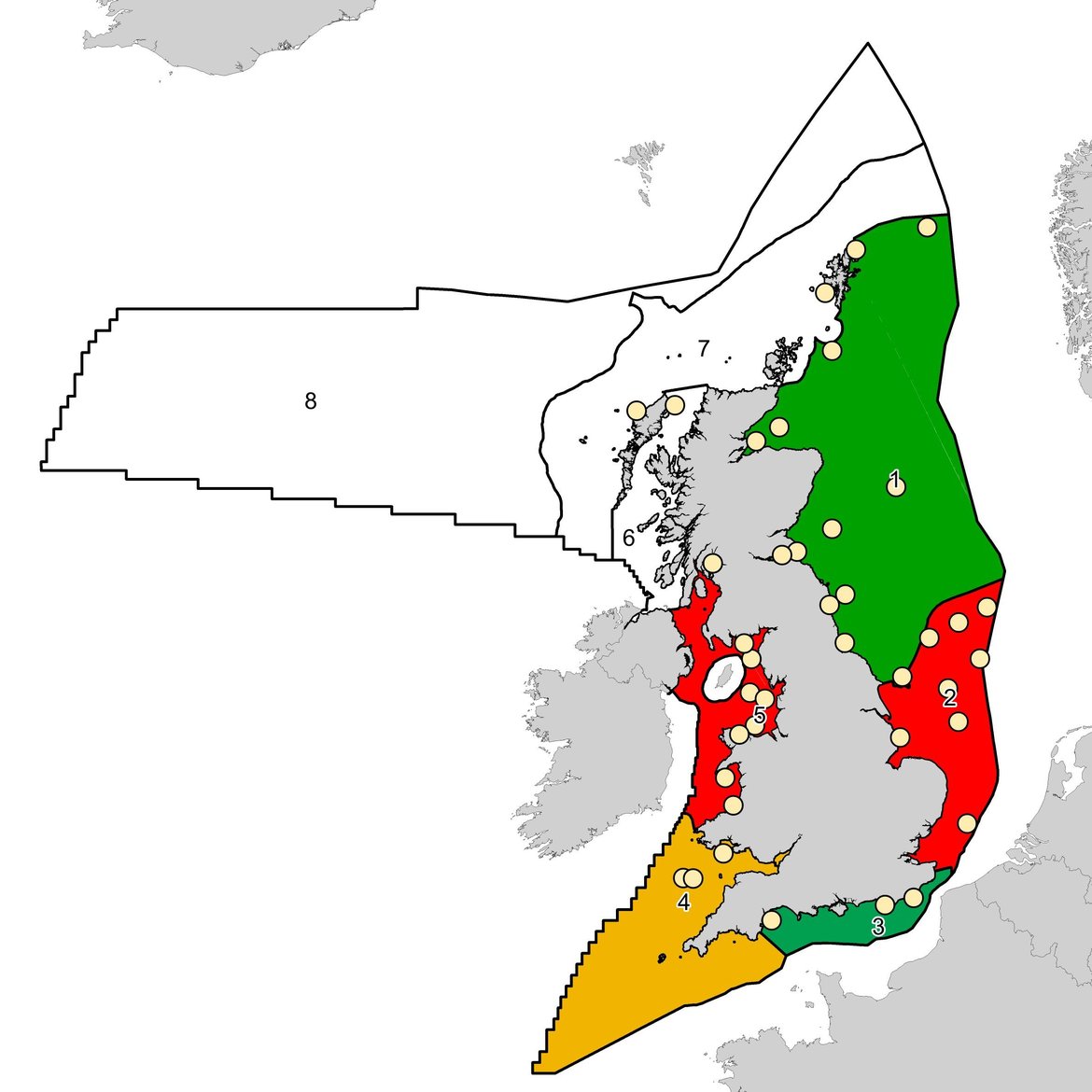
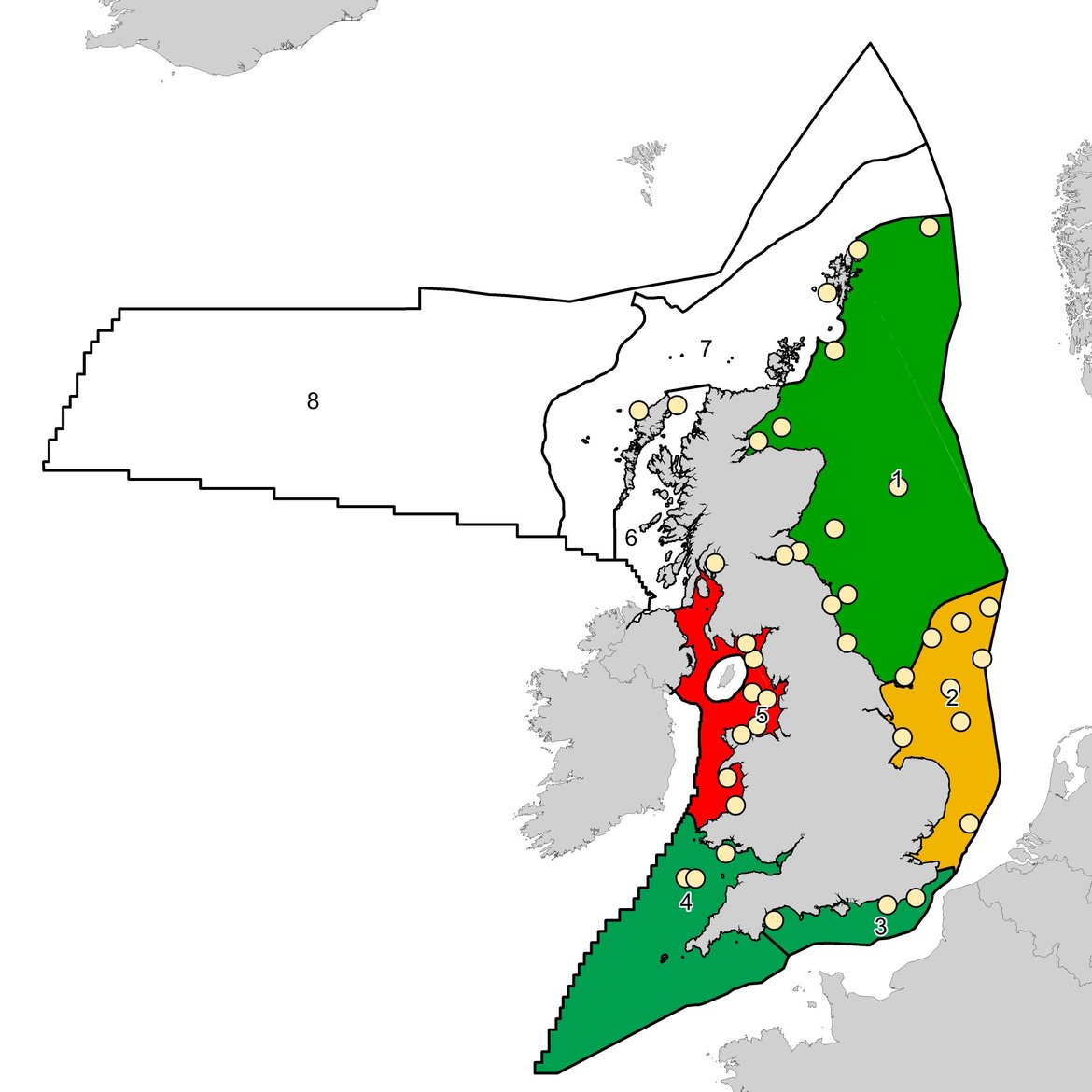
Figure 14: UK biogeographic marine region status for liver neoplasms in dab during (a) Baseline period 2004-2010, and (b) Assessment period 2010-2015. UK marine regions include: (1) Northern North Sea (2) Southern North Sea (3) Eastern Channel (4) Western Channel and Celtic Seas (5) Irish Sea (6) Minches and Western Scotland (7) Scottish Continental Shelf (8) Atlantic Northwest Approaches, Rockall Trough and Faroe/Shetland Channel. Regions coloured red indicate ‘significant response’; amber regions indicate ‘elevated response’; and the green regions indicate a ‘background response’. The grey regions had no data collected. The Northern North Sea and Irish Sea UK marine regions (Regions 1 and 5) are coloured based on England and Wales data only due to the absence of normalised data from Scotland.
UK Marine Region (England and Wales data) | Baseline 2004-2010 (%) | Assessment 2011-2015 (%) | Assessment 2016-2020 (%) |
---|---|---|---|
Eastern Channel | 0,4 | 3,2 | Pending |
Irish Sea | 11,6 | 7,8* | Pending |
Northern North Sea | 2,5 | 1,9* | Pending |
Southern North Sea | 9,1 | 6,0* | Pending |
Western Channel and Celtic Sea | 4,9 | 3,1* | Pending |
Table 4: The percentage prevalence and indicator response threshold assessment of liver neoplasms in analysed populations (3–6-year-old dab) from UK biogeographic marine regions. A ‘*’ denotes a statistically significant change.
This initial assessment uses data from England and Wales, and forms part of a ‘work in progress’ to help develop a liver neoplasm assessment tool for application across the OSPAR Maritime Area. Whilst this case study promising, further work is required to adapt the approach for application across the wider OSPAR Maritime Area. This is determined by the fact that the stocking density and age structure of dab populations across such a large geographical region is likely to vary considerably, meaning the assessment of 3–6-year-old fish from all regions may not be possible. Furthermore, it will be particularly challenging to clearly define indicator response thresholds, or so-called ‘assessment criteria’. It could be argued that the presence of any liver neoplasms is not indicative of a healthy environment and that the prevalence of neoplasms is not only indicative of the toxicity of chemical contaminants but of multiple factors that interact over the life cycle. The prevalence range of liver neoplasms in England and Whales waters is reportedly the highest observed within the OSPAR Maritime Area (Stentiford et al., 2009; Stentiford et al., 2010; Lang et al., 2017). These results should be considered as a critical point of vigilance. Therefore, whilst the separation of this maximum observable range into thirds (as undertaken during this assessment) may initially appear as a rudimentary approach to defining indicator response thresholds, it might serve as a suitable proxy for other regions and inform progress towards establishing good environmental status.
5. Conclusion and perspectives
In a sensitive context of global change and despite the fact that it is technically impossible to monitor each hazardous substance, the assessment of the biological effects of in situ diffuse chemical contamination has progressed significantly since the QSR 2010. Over the past decade, an integrated approach to the biological effects of chemical contaminants has been developed based on chemical and ecotoxicological OSPAR data obtained from organisms collected in their natural habitat.
The selected case studies for the QSR 2023 demonstrate the consensus expertise of eleven countries on a broad geographical scale. Biological response to chemical contamination on cell function as genotoxicity (comet test), neurotoxicity (AChE), oxidant stress, cytotoxicity (Lysosomal stability) in fish and mussels was monitored and also the general health of the organism at the individual level such as the prevalence range liver neoplasms of dab, alters biological functions such as the reprotoxicity of antifouling agent tributyltin on Crangon crangon shrimps populations in the Southern North Sea, and finally increases the frequency of pathologies of benthic fish populations such as dab in the North Sea. The integrated method was divided in 4 steps (Figure 1 and Table 2) and revealed the occurrence of certain heavy metals and organic compounds at concentrations exceeding the EAC thresholds on areas of high chemical pressures (red colour Table 2). For example, mercury bioaccumulation was high (> EAC, red) in almost all sentinel species at all stations. Cadmium and lead are higher in sediment and mussels at most of the sampled stations. Copper is very high in sediment in Denmark and France. Polychlorobiphenyl persistent congener CB118 is particularly concentrated in sediment or in species tissue in Scotland, Ireland and France. PAHs are superior to EAC threshold in the Reykjavik Harbour mussels. The special case study of the specific effects of TBT on shrimp in the sensitive zone of the Southern North Sea representing 90% of the catches in Europe, stresses the ecological and economical importance of expanding the monitoring of imposex to species other than gastropods and initiating an endocrine disrupter monitoring programme.
For the next ten years, two stages of progress should now be achieved: (1) extension of monitoring of chemical contaminants by measuring biological effects under CEMP and (2) drafting guidelines on common biological effects and defining quality assurance with the neighbouring facades of the Arctic, the Mediterranean and the Baltic Sea. Finally, in a future perspective of assessment of chemical exposome in the ocean and the Marine strategy framework, an evolution of the monitoring of biological effects could be carried out (i) by integrating new sentinel species of fish, invertebrate, benthic macrofauna, marine mammals and birds (ii) in developing new bioassays to assess sediment and water toxicity (iii) and integrating new analytical methodologies in ecotoxicology (i.e. omics, immunotoxicity, endocrine disruption and life development) and chemistry (non target screening and new passive samplers on emerging contaminants) for the assessment of biological effects of the chemical contaminants on marine populations.
6. References
Burgeot T., Akcha F., Ménard D., Robinson C., Loizeau V., Brach-Papa C., Martìnez-Gómez C., Le Goff J., Budzinski H., Le Menach K., Cachot J., Minier C., Broeg K., Hylland K., (2017). Integrated monitoring, of chemicals and their effects on four sentinel species, Limanda limanda, Platichthys flesus, Nucella lapillus and Mytilus sp., in Seine bay: A key step towards applying biological effects to monitoring. Marine Environmental Research. 124, 92.105.
Davies, I.M., Vethaak A.D., 2012. Integrated marine environmental monitoring of chemicals and their effects. ICES cooperative research report. N° 315, November 2012. 277p
Dauvin, J.C., Ruelleta, T., Desroy, N., Jansona, A.l., 2007. The ecological quality status of the Bay of Seine and the Seine estuary: Use of biotic indices. Marine Pollution Bulletin Volume 55, Issues 1-6, 2007, Pages 241-257. Available at: http://dx.doi.org/10.1016/j.marpolbul.2006.04.010.
Hawkins, W.E., Walker, W.W., Overstreet, R.M., Lytle, J.S. and Lytle, T.F., 1990. Carcinogenic effects of some polycyclic aromatic hydrocarbons on the Japanese medaka and guppy in waterborne exposures. Science of the total environment, 94(1-2), pp.155-167.
Hylland, K., Robinson, C.D., Burgeot, T., Martìnez-Gómez C., Lang T., Svavarsson J., Thain J.E., Vethaak A.D., (2017a). Integrated chemical and biological assessment of contaminant impacts in selected European coastal and offshore marine areas. Marine Environmental Research. 124, 130-138
Hylland, K., Burgeot, T., Martìnez-Gómez C, Lang T., Robinson C.D, Svavarsson J., Thain J.E., Robinson C.D, Gubbins M.J. (2017b). How can we quantify impacts of contaminants in marine ecosystems? The ICON project. Marine Environmental Research. 124, 2-10
Hylland, K., Bellas,J., Giltrap, M., Brooks,S. 2021. Ices viewpoint background document: How can we quantify and manage the impact of chemical pollution in the oceans? (Ad Hoc). ICES scientific Reports.3:102.29pp. Available at: https://doi.org/10.5/ices.pub.5446.
ICES. 2011. Report of the Study Group on Integrated Monitoring of Contaminants and Biological Effects (SGIMC), 14–18 March 2011, Copenhagen, Denmark. ICES CM 2011/ACOM:30. 265 p
ICES 2014. Report of the Working Group on Crangon Fisheries and Life History (WGCRAN) ICES CM 2014/SSGEF:08. Hamburg: ICES, 40.ICES. 2021. Working group on biological effects of contaminants (WGBEC). ICES Scientific Reports.3:65.90pp. Available at: https//doi.org/10.17895/ices.pub.8222
Judson R., Richard A., Dix D.J., Houck K., Martin M., Kavlock R., Dellarco V., Henry T., Holderman T., Sayre P., Tan S., Carpenter T., Smith E. (2009) The toxicity data landscape for environmental chemicals. Environ Health Perspect. 117(5):685-95. Available at: doi: 10.1289/ehp.0800168.
Lang, T., Feist, S.W., Stentiford, G.D., Bignell, J.P., Vethaak, A.D. and Wosniok, W., 2017. Diseases of dab (Limanda limanda): Analysis and assessment of data on externally visible diseases, macroscopic liver neoplasms and liver histopathology in the North Sea, Baltic Sea and off Iceland. Marine environmental research.
Luttikhuizen, P. C., Campos, J., van Bleijswijk, J., Peijnenburg, K. T. C. A., and van der Veer, H. W. (2008). Phylogeography of the common shrimp, Crangon crangon (L.) across its distribution range. Mol. Phylogenet. Evol. 46, 1015–1030. Available at: https://doi.org/10.1016/j.ympev.2007.11.011.
Mauffret A., Wessel N., Roubeix V., Akcha F., Chouvelon T., Aminot Y., Munschy C., Couteau J., Briaudeau T., Izagirre U., Mahe K., Godfrin Y., Pollono C., Moisan K., Olivier N., Bely N., Bruzac S., Crochet S., Thomas B., Sireau T., Burgeot T. (2021). Campagne dédiée à l'évaluation des effets biologiques induits par la contamination chimique en baie de Seine. SELISEINE 2018. Rapport final. 98p. Available at: https://archimer.ifremer.fr/doc/00688/80024/
MIME 2019. Working Group on Monitoring and on Trends and Effects of Substances in the Marine Environment (MIME). Copenhagen: 18–22 November 2019. OSPAR Convention for the Protection of the Marine Environment of the North-East Atlantic. MIME 19/10/1. 23pp
Myers, M.S., Landahl, J.T., Krahn, M.M., Johnson, L.L. and McCain, B.B., 1990. Overview of studies on liver carcinogenesis in English sole from Puget Sound; evidence for a xenobiotic chemical etiology I: pathology and epizootiology. Science of the Total Environment, 94(1), pp.33-50.
Myers, M.S., Stehr, C.M., Olson, O.P., Johnson, L.L., McCain, B.B., Chan, S.L. and Varanasi, U., 1994. Relationships between toxicopathic hepatic lesions and exposure to chemical contaminants in English sole (Pleuronectes vetulus), starry flounder (Platichthys stellatus), and white croaker (Genyonemus lineatus) from selected marine sites on the Pacific Coast, USA. Environmental Health Perspectives, 102(2), p.200.
Myers, M.S., Johnson, L.L., Hom, T., Collier, T.K., Stein, J.E. and Varanasi, U., 1998. Toxicopathic hepatic lesions in subadult English sole (Pleuronectes vetuls) from Puget Sound, Washington, USA: relationships with other biomarkers of contaminant exposure. Marine Environmental Research, 45(1), pp.47-67.
Parmentier K. F.V., Y. Verhaegen, Els M. Monteyne, B. P. De Witte, Daan H.R. Delbare, P. Roose, G. Smagghe, K. Hylland, T. Burgeot, K. Cooreman 2020. Quality status of the environment: impact of tributyltin on the crustacean Crangon crangon during and following legislative actions. New challenge in marine pollution monitoring. Frontiers in Marine Science, 6(633), 14p. Publisher's official version: https://doi.org/10.3389/fmars.2019.00633
Roose P., Albaigés J., Bebianno M.J.,Camphuysen C., Cronin M., de Leeuw J., Gabrielsen G., Hutchinson T., Hylland K., Jansson B., Jenssen B.M., Schulz-Bull D., Szefer P., Webster L., Bakke T., Janssen C. (2011). Chemical Pollution in Europe’s Seas: Programmes, Practices and Priorities for Research, Marine Board Position Paper 16. Calewaert, J.B. and McDonough N. (Eds.). Marine Board-ESF, Ostend, Belgium
Stehr, C.M., Myers, M.S., Johnson, L.L., Spencer, S., Stein, J.E., 2004. Toxicopathic liver lesions in English sole and chemical contaminant exposure in Vancouver Harbour, Canada. Marine Environmental Research 57, 55-74.
Stentiford, G.D., Bignell, J.P., Lyons, B.P. and Feist, S.W., 2009. Site-specific disease profiles in fish and their use in environmental monitoring. Marine Ecology Progress Series, 381, pp.1-15.
Stentiford, G.D., Bignell, J.P., Lyons, B.P., Thain, J.E., Feist, S.W., 2010. Effect of age on liver pathology and other diseases in flatfish: implications for assessment of marine ecological health status. Marine Ecology Progress Series 411, 215-230.
Verhaegen, Y. (2012). Mechanism, Concentrations and Effects of Tributyltin in Common Shrimp Crangon crangon. PhD. Dissertation. Ghent University: Ghent.
Verhaegen, Y., Monteyne, E., Neudecker, T., Tulp, I., Smagghe, G., Cooreman, K., et al. (2012). Organotins in North Sea brown shrimp (Crangon crangon L.) after implementation of the TBT ban. Chemosphere 86, 979–984. Available at: https://doi.org/10.1016/j.chemosphere.2011.11.028
Vethaak, A.D., Jol, J.G. and Pieters, J.P., 2009. Long-term trends in the prevalence of cancer and other major diseases among flatfish in the southeastern North Sea as indicators of changing ecosystem health. Environmental science & technology, 43(6), pp.2151-2158.
Abbreviations
Coordinated Environmental Monitoring Programme (CEMP), Monitoring and on Trends and Effects of substances in the Marine Environment (MIME), Environmental assessment criteria (EAC), Background assessment concentration (BAC), Marine Strategy Framework Directive (MSFD), Good environmental status (GES), Integrated Assessment of Contaminant Impacts on the North Sea (ICON), Monitoring of Biological Effects Induced by Chemical Contamination (SELI), hexabromocyclododecane (HBCDD), Polycyclic aromatic hydrocarbons (PAHs), polychlorobyphenyls (PCBs), Polybrominated diphenyl ethers (PBDEs), Organoclorine pesticides(OCPs).
Contributors
Lead authors - Thierry Burgeot and Aourell Mauffret
Supporting authors - Steven Brooks, Ketil Hylland, Joachim Sturve, Lars Förlin, John Bignell, Jörn Scharsack, Hannah Anderson, Kris Coorman, Koen Parmentier, Marta Assuncao, Michelle Giltrap, Brendan McHugh, Halldor Palmar Halldorsson, Hermann Dreki Guls, Zhanna Tairova, Concepcion Martinez-Gomez, Juan Antonio Campillo and Juan Bellas Bereijo
Supported by: Monitoring and on Trends and Effects of Substances in the Marine Environment Working Group (MIME), the Working Group on Biological Effects of Contaminants (ICES WGBEC) and the Hazardous Substances and Eutrophication Committee.
Citation
Burgeot, T., Mauffret, A., J., Anderson, Brooks, S., Assuncao, M., Bellas Bereijo, J., Bignell, J., Campillo, J.A., Coorman, K., Förlin, L., Giltrap, M., Guls, H.D., Hylland, K., Halldorsson, H.P., Martinez-Gomez, C., McHugh, B., Parmentier, K., Scharsack, H., Sturve, J. and Tairova, Z. 2022. Integrated biological effects and chemical contaminants approach: a case study. In: OSPAR, 2023: The 2023 Quality Status Report for the North-East Atlantic. OSPAR Commission, London. Available at: https://oap.ospar.org/en/ospar-assessments/quality-status-reports/qsr-2023/other-assessments/integrated-biological-effects