Feeder Report 2021 - Offshore Renewable Energy Generation
Distribution, Intensity and Trends since QSR 2010
Overall trends - Offshore wind
2.1 QSR 2010 noted that energy production by offshore wind farms had emerged as a new use of coastal and shallower offshore waters over the previous decade. In 2009, 17 wind farms, covering over 500 km2 and involving 713 turbines, were operational or under construction, giving a total capacity of almost 1 900 MW. All development was in the Greater North Sea and the Celtic Seas.
2.2 The following decade saw substantial growth in offshore wind. Parts of the OSPAR Maritime Area are very favourable for offshore wind development. The shallow waters of the North Sea have high and widespread potential, and the North Sea is the world’s leading region for deployed offshore wind capacity. The Atlantic Ocean has a high natural potential for both bottom-fixed and floating offshore wind energy. According to the European Commission, the United Kingdom, Denmark and Germany are the clear leaders in offshore wind development in Europe (European Commission, 2020a; 2020b).
2.3 By the end of 2019, there was 22 072 MW of installed offshore wind capacity in Europe, produced by over 5 000 turbines (WindEurope, 2020). Almost all – 99% - of this capacity was in five countries: the United Kingdom (45% of all installations), Germany1 (34%), Denmark (8%), Belgium (7%) and the Netherlands (5%). OSPAR countries with small amounts of offshore wind generation2 included Spain, France, Sweden, Norway, Ireland and Portugal. The North Sea accounted for 77% of Europe’s installed capacity, and the Irish Sea 13%3. A net new offshore wind capacity of 3 632 MW of offshore wind capacity was added in 2019, the highest annual increase ever, nearly half of which was in the United Kingdom. By the end of 2019, Europe accounted for around 75% of global offshore wind capacity installed (European Commission, 2020c).
Number of wind farms connected | Number of turbines connected | Cumulative capacity (MW) | |
---|---|---|---|
United Kingdom | 40 | 2 225 | 9 945 |
Germany | 28 | 1 469 | 7 445 |
Denmark | 14 | 559 | 1 703 |
Belgium | 8 | 318 | 1 556 |
Netherlands | 6 | 365 | 1 118 |
Sweden | 5 | 80 | 192 |
Ireland | 1 | 7 | 25,2 |
Spain | 2 | 2 | 5 |
Portugal | 1 | 1 | 8,4 |
Norway | 1 | 1 | 2,3 |
France | 1 | 1 | 2 |
Figure 1 Route density map of service vessels in the OSPAR area 2019 (Source EMODnet 2020) and the Locations of Offshore Renewable Energy Developments https://odims.ospar.org/maps/1820/
2.4 The average rated capacity of turbines, the size of wind farm and the distance to shore have increased. The average size of windfarms in construction almost doubled over the decade, to 621 MW; the average capacity of a turbine installed in 2019 was 7,8 MW, 1 MW larger than in 2018 and more than double the average size in 2010 of 3,2 MW; and the average distance of new installations to shore (59 km) and water depth (33 m) continued to increase, even though most wind farms are bottom-fixed (WindEurope, 2011; WindEurope, 2020). The costs of offshore wind have fallen steadily over the past ten years. In the second half of the decade, costs decreased from over €200/MWh in 2014 to a range of €45-79/MWh at the end of 2019. Drivers for the reduction include upscaling of turbine size, economies of scale due to larger project size, weight reduction due to innovative materials, and favourable financing (European Commission, 2020b; 2020c).
2.5 Floating offshore wind is at an earlier stage of development. The European Union’s (EU) Blue Economy Report 2020 stated that only 45 MW of the total European (including the United Kingdom) offshore wind capacity in 2019 was from this source (European Commission, 2020a). The report also identified over 300 MW of additional capacity planned to be operational by 2024, in the United Kingdom, Norway, France, Spain and Portugal. Various technologies are in development. Capacity in 2019 involved different floating substructures: spar-buoys (the 30 MW Hywind project in Scotland); barges (the 2 MW Floatgen project in France) and semi-submersibles (the 25 MW WindFloat Atlantic project in Portugal, partially operational in 2019).
Overall trends – ‘wet renewables’
2.6 So-called ‘wet renewables’ harness energy from tidal currents and wave power. Within the OSPAR Maritime Area, the Atlantic Ocean has good natural potential for wave and tidal energy, and the North Sea also has localised potential (European Commission, 2020a).
2.7 Advice to OSPAR from the International Council for the Exploration of the Sea (ICES) in 2019 summarised the types and distribution of tidal and wave energy instillations, potential future trends, and environmental impacts (ICES, 2019). Key messages from the advice are summarised briefly in sections 2, 4 and 6 of this feeder report. Tidal stream resource in selected European countries is shown below:
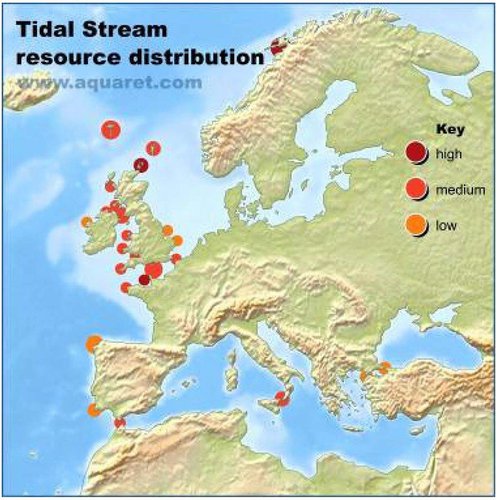
Figure 2 Tidal stream resource distribution in Europe evaluating the tidal current resource for selected locations around Europe, with predefined characteristics making them suitable for tidal stream energy exploitation. Taken from ICES 2019, original source: www.aquaret.com
2.8 ICES identified 43 MW of tidal energy devices operational across the OSPAR area, with more than 320 MW under construction, consented or in planning phases. Tidal energy installations include different types of turbine, hydrofoils, Archimedes screws and tidal kites. In the OSPAR area, the United Kingdom had the largest level of deployment of tidal energy, with test centres as well as commercial sites under development. ICES noted that operational or planned developments also existed in France, Belgium, the Netherlands, Norway and Spain4.
2.9 Tidal energy can also be obtained using barrages across estuaries, or by enclosing a tidal lagoon. The long-established barrage at La Rance in France was the world’s first tidal power station and has a peak capacity of 240 MW; however, there are no current plans for further development of this form of tidal energy in France (French Government, 2019). ICES noted that environmental concerns pose serious constraints on new tidal energy barrages in the OSPAR area, although installation of turbines in existing coastal infrastructure remains an attractive option.
2.10 In the case of wave energy, ICES reported that the OSPAR countries best suited for the technology are the United Kingdom, Ireland and Norway, followed by northern Spain, France and Portugal.
2.11 A coastline infrastructure energy plant – turbines housed in a breakwater - with a capacity of 300 kW is operational in northern Spain, and a range of devices are being trialled within the OSPAR area. However, excluding test centres, less than 1 MW of wave energy was operational across the OSPAR area at the time of the ICES report, with around 20 MW consented or in planning phases. To date, there are no operational commercial-scale nearshore fixed and floating wave energy developments within the OSPAR area.
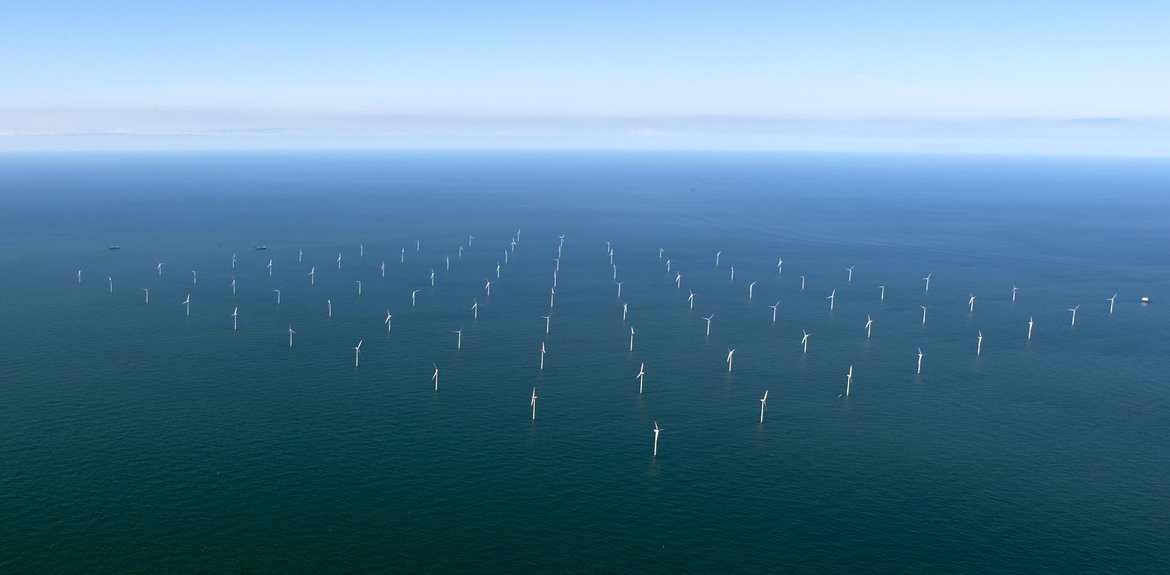
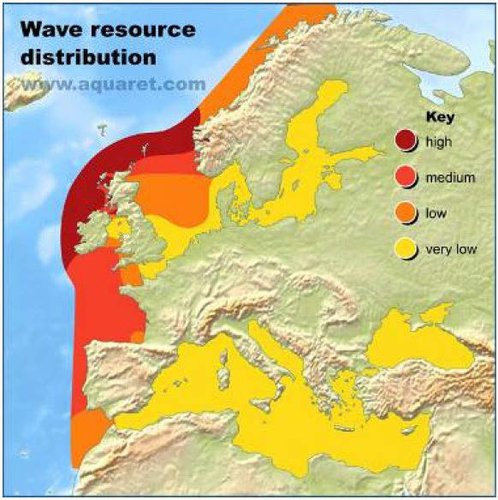
Figure 3 Wave energy resource distribution in Europe. Taken from ICES 2019, original source www.aquaret.com
Economic value
3.1 The EU’s Blue Economy report 2020 included partial economic data for the EU offshore wind energy (production and transmission) sector in 20185 (European Commission, 2020a). Specifically:
- the sector directly employed 4 624 persons, up from 582 in 2009. The largest number was in the United Kingdom (2 758 persons), followed by Denmark (767), the Netherlands (743), and Belgium (356);
- the gross value added in the United Kingdom was €521 million, in Denmark €463 million, and in Belgium €105 million;
- net investment and turnover were highest in the United Kingdom, gross profit was highest in Denmark.
3.2 The wider supply chain for offshore wind creates employment more widely. For example, the Blue Economy report cited an estimate of 27 000 jobs created in Germany due to the development of offshore wind. This employment extends beyond coastal regions to manufacturing facilities elsewhere: for example, key turbine components are made in southern and western Germany (European Commission, 2020a). The 2020 EU strategy on offshore renewable energy stated that the offshore wind industry in the European Union employs 62 000 people (European Commission, 2020b).
3.3 The economic status of tidal and wave energy, given its current state of development, is far smaller than offshore wind. Employment in the sector in the European Union is around 2 500 people (European Commission, 2020b).
3.4 In global terms, OSPAR countries are global leaders in offshore renewable technologies and industries, based on first-mover status for offshore wind turbines and a strong home market. Around 42% of the global market in cumulative installed capacity is in the EU and another third is in the United Kingdom. Exports in the wind sector (both onshore and offshore) from the EU28 increased steadily between 2009 and 2018, to €2,32 billion, a 47% share of global exports. Imports during that period were far smaller, ranging between €0,03 billion and €0,17 billion (European Commission, 2020c). European countries are also leaders in the new technologies of floating wind and of tide and wave energy (European Commission, 2020b), or may have transferable expertise from other industries, such as petroleum (Norwegian Ministries, 2019).
3.5 Major investment is associated with future ambitions for increasing offshore renewable generation (see section 4 below). For example, the European Commission gives a figure of up to €800 billion needed to meet objectives for 2050, and the United Kingdom Government has stated that its ambitions for offshore wind could encourage £20 billion of private investment into the United Kingdom and double jobs in the sector over the next decade (UK Government, 2020a).
Future trends
4.1 A further major expansion of offshore renewable energy is anticipated in the OSPAR Maritime Area in the coming decade and beyond, primarily of offshore wind but also involving tidal and wave power. There may also be developments of other novel technologies.
4.2 Co-location opportunities of marine renewable energy infrastructure with other uses are being investigated, in particular in relation to offshore shellfish culture. The Edulis project in Belgium has trialled offshore mussel culture in a wind farm, and the potential for using wind farms for flat oyster restoration projects has been studied in the Netherlands (European Commission, 2020e).
Future trends – offshore wind
4.3 The European Commission’s 2020 strategy on offshore renewable energy envisages an expansion in offshore wind from the current installed capacity of around 12 GW in the EU27 to at least 60 GW by 2030 and 300 GW by 2050 (European Commission, 2020b). In the short term, most of this will be through bottom-fixed installations, but floating offshore wind is expected to develop further. Nearly 80% of the wind in Europe blows in waters that are at least 60 m deep and are thus unsuitable for bottom-fixed installations on grounds of cost. The estimated technical potential for floating offshore wind in Europe is around 4 540 GW, 3 000 GW of which is in water depths between 100 m and 1000 m (European Commission, 2020a)6 . The Commission’s strategy anticipates that around 150 MW of floating offshore wind will be commissioned by 2024, and that there is potential to reduce the costs of electricity from floating installations to below €100/MWh by 2030.
4.4 The strategy identifies various challenges associated with the expansion to 2050, and ways to address them. These include effective maritime spatial planning, ensuring coexistence with other uses of the sea, as well as compliance with environmental legislation (see section 6 below). While the strategy states that expansion of offshore energy is not incompatible with shipping routes, it also notes the importance of risk management, given that the areas with most potential for offshore renewable energy are the most exposed to tensions with other uses, such as risks of collisions with vessels, fishing gear, military activities, or dumped ammunitions and chemicals. Other areas to address include grid planning, the energy market framework (more on market arrangements is at European Commission, 2020d), EU financing mechanisms, and research and development. Regional cooperation in sea basins is highlighted, such as through the existing North Seas Energy Cooperation (NSEC) mechanism (NSEC, 2020).
4.5 The Commission’s strategy highlights the potential for wind energy in different sea basins, but does not specify where the increase in European installed capacity will occur in the next decade, or beyond. Precise locations will depend on factors such as available space, sea depths, wind speeds, cost, and where energy demand is located. However, substantial expansion in the next decade can be expected in OSPAR waters. For example, NSEC states7 :
- for the North Seas, the ministerial meeting of NSEC in 2019 agreed to work together to achieve an indicative aggregate installed capacity of EU Member States of NSEC of at least 70 GW by 20308 (NSEC, 2020);
- the Netherlands is planning for an overall capacity of 11 GW by 2030, involving 9,6 MW of new capacity being commissioned between 2020 and 2029 (Netherlands Ministry of Economic Affairs and Climate Policy, 2020);
- Belgium plans for around 4 GW of capacity by 2030, almost double the capacity in 2020 (Belgian Government, 2019);
- Denmark is considering the possibility of building an ‘energy island’ in the North Sea with at least 10 GW of offshore wind connected by 2030 (Danish Ministry of Climate, Energy and Utilities, 2019);
- in France, the Government set a target of 5,2-6,2 GW of capacity by 2028, compared with 0,5 GW a decade earlier (French Government, 2019);
- Germany projects an increase in offshore wind capacity to around 20 GW by 2030, from less than 8 GW in 2020 (German Federal Government, 2019); in November 2020, the Bundestag adopted a target of 40 GW by 2040 (Deutscher Bundestag, 2020);
- Ireland is targeting at least 3,5 GW of offshore renewable energy by 2030, mainly of offshore wind (Irish Department of Communications, Climate Action and Environment, 2019).
4.6 Smaller expansion is expected to 2030 in Portugal, to just 0,3 GW, although this is a ten-fold increase on the current capacity. Exploring the potential of offshore wind is a priority for the next decade (Portuguese Government, 2019). In Spain, a strategy for the development of offshore wind is being developed (Spanish Government, 2019).
4.7 In the United Kingdom, the United Kingdom Government announced in 2020 an ambition to quadruple the amount of offshore wind to a capacity of 40 GW by 2030, including 1 GW of innovative floating offshore wind. This expansion could support up to 60 000 jobs in 2030 (UK Government, 2020a). Within the United Kingdom, the Scottish Government’s offshore wind policy statement states that as much as 11 GW of installed capacity is possible by 2030, compared with less than 1 GW installed and operational in 20209 . Further major expansion is expected after 2030 (Scottish Government, 2020a). A sectoral plan for offshore wind energy outlines areas for development (Scottish Government, 2020b).
4.8 Other non-EU countries within the OSPAR Maritime Area have small expansion plans in the next decade. In Norway, cost trends will determine how far offshore wind will compete with land-based alternatives, but the Government has opened two areas for licence applications (Norwegian Ministries, 2019; 2020). A 96-120MW offshore wind farm is currently planned for the Faroe Islands, with the aim of being operational by 2025 (SEV, 2020b).
4.9 Overall, most of the expansion of offshore wind in European seas to 2030 and beyond will be in the OSPAR Maritime Area. Under a maximum scenario developed by the wind industry of 450 GW installed in European seas10 by 2050, 212 GW would be expected in the North Sea11 , 85 GW in the Atlantic and Irish Sea off France, Ireland and the UK, and 22 GW in Portuguese and Spanish waters12 (WindEurope, 2019; also referred to in European Commission, 2020e). An additional 83 GW was projected for the Baltic.
4.10 The 380 GW of offshore wind projected for the northern seas (including the Baltic) would require 76 000 km2 of sea space (assuming 5 MW/km2), around 2,8% of the total area of the northern seas, and approximately equivalent to the size of the island of Ireland (European Commission, 2020e). However, in some regions the proportion of the sea area occupied by offshore wind would be far higher – for example, 15-20% in parts of the southern North Sea, and 5-15% in the Irish Sea (WindEurope, 2019).
Future trends – wet renewables
4.11 The current scale of tidal and wave energy installations is small. Nevertheless, some increase in the next decade can be expected. ICES (2019) advised that wet renewables will be increasingly installed in the marine environment in the near future, and that the large number of developments in planning stages suggests a strong industry-led potential for increasing developments. For tidal devices, the trend seems to be away from heavy bottom mounted devices and towards floating tidal devices, which are easier to deploy and maintain, cheaper, and tap into faster tidal flows. For tidal lagoons, significant economic and environmental challenges remain although there is still interest in developing the technology. In the case of wave energy, ICES reported continuing test programmes at sites in the United Kingdom, Spain, France, Norway and Ireland.
4.12 Factors which could increase the opportunity for future developments include new marine spatial planning support tools, better understanding of the environmental impact of marine renewables, and new technologies. Nonetheless, many past applications have been withdrawn due to financial or logistical issues, and barriers to overcome include survivability and reliability of installations, uncertainties about environmental impacts, and investment conditions (ICES, 2019).
4.13 European Union analysis has reached similar conclusions. While the current scale of installations is only a small fraction of offshore renewable energy (see paragraph 2.8), the potential for wave and tidal energy in the EU is said to be vast, with a theoretical annual potential of wave energy of about 2 800 TWh, and for tidal current of about 50 TWh (European Commission, 2020a). The Commission’s offshore renewable energy strategy aims for at least 1 GW of ocean energy capacity by 2030, with a view to 40 GW by 2050 (European Commission, 2020b).
4.14 At present, there is no dominant technology for tidal and wave energy generation, and significant cost reductions would be needed for them to play a significant role in the energy mix. There has been some drop in costs in recent years: by 2020 costs had fallen by 40% since 2015. A key step towards commercial uptake would be to implement an existing programme of 100 MW pilot projects by 2025 (European Commission, 2020b).
Other energy sources
4.15 Other offshore energy technologies exist, but are in relatively early stages of research and demonstration. Floating solar photovoltaic installations may have applications in industries such as aquaculture, or for remote coastal communities. Sea trials of a 17 kW system have demonstrated its survivability in storm conditions. Offshore hydrogen generation is being investigated in combination with tidal turbine systems or offshore wind (European Commission, 2020a).
Quality Status Report 2010 and Intermediate Assessment 2017
5.1 QSR 2010 noted that impacts arise throughout the life cycle of wind farms, including site selection, construction, operation, decommissioning and removal. Specific environmental issues highlighted were noise, disturbance and loss of habitats, bird collisions, and visual intrusion, as well as potential impacts on other uses of the sea (e.g. hazards to shipping, displacement of fishing) and conflicts with marine conservation objectives.
5.2 QSR 2010 concluded that knowledge about the environmental impacts of offshore wind was limited, and recommended that OSPAR should address knowledge gaps and keep measures to manage environmental impact under review. The QSR did not discuss other forms of marine renewable energy generation.
5.3 IA 2017 did not cover marine renewable energy specifically, although noise impacts of pile driving during the installation of wind turbines were covered in its assessment of impulsive noise.
Analysis of specific pressures, impacts and measures
6.1 Understanding and managing the environmental impacts of the substantial existing and future growth of offshore renewable energy are essential for its sustainable development. The European Commission’s offshore renewable energy strategy acknowledges that monitoring and scientific knowledge need to be developed, including through in-depth analysis, data exchange and modelling tools, in order to understand potential cumulative effects on the environment and interactions with other activities (European Commission, 2020b). The strategy proposes ways to take this forward, including building a ‘community of practice’ to promote sharing of experience and joint working. The European Commission has also published a revised guidance document on wind energy developments and EU nature legislation (European Commission, 2020e – further details below).
OSPAR measures
6.2 OSPAR produced guidance on environmental considerations for offshore wind farm development in 2008 (OSPAR, 2008). The guidance aimed to assist those involved with developments to identify and consider issues that may be associated with the environmental impacts of developments, covering location, licensing, monitoring, construction and operation, and decommissioning. A 2020 OSPAR survey of its Contracting Parties showed that the offshore wind guidance was generally fully implemented, or that implementation was in progress, although not all Contracting Parties provided information for the survey. OSPAR also maintains a database of individual marine renewable developments, including tidal and wave as well as offshore wind.
Impacts and measures – offshore wind
6.3 The European Commission’s guidance document discusses offshore wind developments in the context of the European Birds and Habitats Directives (European Commission, 2020e). It describes the approach to assessing developments which could affect Natura 2000 sites, species protection provisions, and the role of Strategic Environmental Assessment (SEA) and Environmental Impact Assessment (EIA) procedures. It notes that assessment of cumulative environmental impacts is very relevant to wind farm development, particularly in view of the projected expansion in capacity. Understanding potential cumulative impacts is nevertheless complex: for example, impacts at population level are poorly understood; the extent of pressures is difficult to evaluate; and data availability can be lacking. The guidance includes recommended approaches for dealing with the challenges of cumulative impacts assessment. It also highlights examples of existing good practice, such as from the Netherlands on cumulative impact assessments, and from the United Kingdom on handling uncertainty in design trends. The role of strategic planning, in the context of the Maritime Spatial Planning Directive and the Marine Strategy Framework Directive, is also emphasised. Wildlife sensitivity maps, such as the seabird mapping and sensitivity tool (SeaMaST) developed for English waters, are also a useful tool.
6.4 The Commission’s guidance includes an overview table of potential types of impacts on major offshore receptor groups:
Receptor | Potential impacts of offshore energy developments |
Habitats | Marine habitat loss |
Fish | Electromagnetic fields |
Birds | Habitat loss and degradation |
Marine mammals | Habitat loss and degradation |
Bats | Disturbance and displacement |
Other species | Noise disturbance and displacement |
6.5 Other work highlights the potential scale of change. For example, a report for the Netherlands Government on the system effects of large-scale implementation of offshore wind in the southern North Sea suggests that the upscaling to 2050 is likely to have fundamental impacts on the physical functioning of the North Sea, including on local wind patterns, wave generation, tidal amplitudes, stratification of the water column, dynamics of suspended particles and bedload transport of sediment. These changes may have far-reaching consequences for the ecosystem, including on primary production, food availability across trophic levels, and habitat suitability (Deltares, 2018).
6.6 The Commission’s guidance outlines mitigation methods to address the potential impacts. It recommends appropriate siting of wind installations to avoid impacts on protected habitats and species, and the use of the least disturbing methods for activities such as cable installations. It also notes that the creation of artificial reef habitats on the foundations of structures can affect biodiversity, particularly in areas without natural rock habitats such as large parts of the North Sea. This can increase the biodiversity of benthic habitats, but also affects local community structure. The risk that the foundations may also enable establishment of alien invasive species also has to be considered (European Commission, 2020e).
6.7 The Commission notes the need to consider the potential impacts on fish or marine mammals of noise from offshore wind developments, in particular impulsive noise from pile driving of foundations. Impacts may include physical effects (e.g. damage to hearing) and behavioural effects (e.g. driving animals away from favoured habitats). Increased levels of noise from the operation of wind developments (e.g. noise from maintenance vessel movements) may also have negative impacts. Mitigation measures can include appropriate siting of developments, scheduling of activities to avoid sensitive periods (although hard to implement for species with long sensitive periods, such as harbour porpoise), engineering and surveillance approaches to reduce the risk of noise impacts, and deterrents.
6.8 In the case of birds, the guidance discusses mitigation measures such as design of infrastructure (e.g. to reduce collision risk), scheduling of activities to avoid disturbance during sensitive periods, temporary shutdown of turbines (e.g. during migration periods), and acoustic or visible deterrents.
6.9 The need for improved evidence to support decision-making on offshore wind developments is being addressed in various monitoring and research programmes, including in Belgium (Degraer et al, 2019), the Netherlands (Noordzeeloket, 2020) and the United Kingdom (The Crown Estate, 2020; UK Government, 2020b; UK Offshore Wind Enabling Actions Programme13). Work exploring the use of Bow Tie Analysis to the concept of ecosystem services as a way to assist decision making in OSPAR has also been carried out (Rijkswaterstaat, 2020).
Impacts and measures - wet renewables
6.10 The ICES advice to OSPAR highlighted that because tidal and wave devices remove energy from the marine system, they have the potential to affect local and wider hydrodynamics (ICES, 2019). Significant changes may only occur with large-scale installations, above 1,5 GW. Regional hydrodynamic models can be used to assess the potential impacts of developments, but the uncertainties involved means that continued monitoring of environmental impacts is important.
6.11 Similarly, developments could have physical seabed and sediment transport impacts affecting erosion and deposition of coastlines and offshore sandbanks, or changes to bathymetry or geomorphology. Again, large developments have the highest potential to cause change, but there may also be localised impacts such as scouring.
6.12 ICES advice covered potential impacts on marine life, including the benthos, fish, birds, and marine mammals. In the case of benthos, most changes would be local and site specific, with broader spatial effects mainly associated with tidal barriers or lagoons. Benefits for the benthic habitat can be achieved through design of infrastructure, or through exclusion of bottom fishing. However, colonisation of structures by non-indigenous species could also occur.
6.13 In the case of fish, siting of structures should avoid essential habits such as nursery zones, spawning grounds, and migration routes. Impacts on foodwebs (e.g. due to fish aggregation), collision risks, effects of cables (e.g due to electro-magnetic fields), and behavioural responses to noise could occur, but require further research and monitoring. Collision risk and habitat displacement are potential concerns for mammals and birds; underwater noise could also be a concern.
6.14 ICES highlighted the need for strategic research and monitoring to improve understanding of impacts on key receptors, and to facilitate a more ecosystem level approach to cumulative impact assessments. ICES suggested development of guidance and examples of cumulative impact assessment approaches. The potential effects of other emerging technologies needed to be kept under review, as well as analysis of decommissioning options.
Conclusions
7.1 Key messages14
- The scale of offshore wind installation in the OSPAR Maritime Area is expected to increase greatly in the next decade and beyond, primarily in the Greater North Sea and the Celtic Seas;
- Knowledge on ecological impacts has grown over the last decade, but the evidence and data are still uncertain, particularly in relation to cumulative effects of this major expansion. Major evidence programmes to address gaps in understanding are in progress, and OSPAR may wish to engage with these;
- Growth in tidal, wave and floating solar energy generation over the next decade is likely to be small, but OSPAR may wish to keep in touch with work to increase understanding of potential environmental impacts and mitigation measures.
Distribution and intensity of activity
7.2 At the end of 2019, around 75% of global offshore wind energy capacity was installed in European seas, notably in the Greater North Sea and the Celtic Seas. 77% of European capacity was in the North Sea and 13% in the Irish Sea. Offshore wind installation in other OSPAR Regions is very small in comparison. (Paragraphs 2.1 – 2.5)
Renewable energy production from tidal and wave energy devices is largely at an early and experimental stage. (Paragraphs 2.6 – 2.11)
Trends
7.3 The amount of offshore wind energy capacity has increased substantially since QSR 2010; the size of turbines, size of wind farms and average distance to shore have also increased. (Paragraphs 2.1 – 2.5)
Accelerated expansion of offshore wind energy is anticipated in the next decade, with installed capacity in 2030 several times higher than in 2020. Further expansion is planned after 2030. Expansion to 2030 will primarily be of fixed turbines, but floating installations also has potential in the longer term. (Paragraphs 4.3 – 4.10)
Some increase in wave and tidal energy installations, including pilot projects, is anticipated to 2030, but significant cost reductions would be needed for these to play a significant role in energy generation. (Paragraphs 4.11 – 4.14)
Economic value
7.4 European countries are among the world leaders in offshore energy technology, and the supply chain extends to manufacturing facilities beyond coastal regions. Major investment is associated with ambitions for offshore renewable energy expansion; for example, the European Commission gives a figure of up to €800 billion needed to meet objectives for 2050. (Paragraphs 3.1 – 3.5)
Pressures and impacts
7.5 Offshore renewable energy installations have impacts on many components of the marine environment, including habitats, birds and marine mammals. Uncertainty remains about the nature and scale of impacts, including cumulative effects. Several research and evidence programmes are seeking to improve understanding of the environmental impacts. (Paragraphs 6.1 – 6.14)
Measures
7.6 Mitigation measures such as appropriate siting, management of installation procedures, and turbine design are used to address potential impacts of offshore wind energy. (Paragraphs 6.3 – 6.8)
Summary table
7.7 (Assessment is for offshore wind.)
OSPAR REGIONS15 | |||||
I | II | III | IV | V | |
Relative intensity16 | L | H | M | L | L |
Trend since 2010 | ↑ | ↑ | ↑ | ↑ | ↔ |
Forecast trend to 203017 | ↑ | ↑ | ↑ | ↑ | ↔ |
Confidence assessment for future trends | Very high | Very high High Medium Low Very low | Very high High Medium Low Very low | Very high High Medium Low Very low | Very high High Medium Low Very low |
Footnotes
1Including the Baltic for Germany and Denmark
2Including non-OSPAR waters
3The Baltic accounted for most of the other European capacity (10%)
4A tidal energy installation, using a kite system, is also under development in the Faroe Islands (SEV 2020a).
5Figures for Germany were not available and only employment and investment data were available for the Netherlands (European Commission, 2020a)
6France estimates a technical capacity of 50 GW in relation to its waters, after taking account of other uses
7Estimates from NSEC, Denmark, France, and Germany include OSPAR and non-OSPAR waters
8Aggregated planned project pipeline by EU Member States, including the United Kingdom at that time, of NSEC (including for offshore areas other than the North Seas)
9The total offshore wind capacity consented in Scotland as at 2020 was over 5 GW
10Including non-EU waters
11Including all Norwegian waters
12Including the Mediterranean and Macronesia
13Initiated in Autumn 2020, the Offshore Wind Enabling Actions Programme is a three-year programme designed to increase understanding of the environmental impacts of offshore wind and find strategic solutions to manage and mitigate impacts in order to reduce barriers to the expansion of offshore wind in English waters.
14The views expressed on key messages are those of the assessor and do not necessarily represent the views of the OSPAR Commission
15For the delineation of OSPAR regions see https://www.ospar.org/convention/the-north-east-atlantic
16Low/medium/high
17Trend is in capacity installed
References
Belgian Government 2019. National energy and climate plan. Available at: https://ec.europa.eu/energy/sites/ener/files/documents/be_final_necp_parta_en.pdf
Danish Ministry of Climate, Energy and Utilities 2019. Denmark’s integrated national energy and climate plan. Available at: https://ec.europa.eu/energy/sites/ener/files/documents/dk_final_necp_main_en.pdf
Degraer, S., Brabant, R., Rumes, B. & Vigin, L. (eds). 2019. Environmental Impacts of Offshore Wind Farms in the Belgian Part of the North Sea: Marking a Decade of Monitoring, Research and Innovation. Brussels: Royal Belgian Institute of Natural Sciences, OD Natural Environment, Marine Ecology and Management, 134 p. winmon_report_2019_final.pdf (naturalsciences.be)
Deltares (2018). Assessment of system effects of large-scale implementation of offshore wind in the southern North Sea. Available at: https://www.noordzeeloket.nl/publicaties/
Deutscher Bundestag 2020. Bundestag beschleunigt den Ausbau von Windenergie auf. Available at: https://www.bundestag.de/dokumente/textarchiv/2020/kw45-de-windenergie-see-802490
European Commission 2020a. The EU blue economy report 2020. Publications Office of the European Union. Luxembourg. Available at: https://blueindicators.ec.europa.eu/published-reports_en
European Commission 2020b. An EU Strategy to harness the potential of offshore renewable energy for a climate neutral future. COM(2020) 741 final. Available at: https://ec.europa.eu/energy/sites/ener/files/offshore_renewable_energy_strategy.pdf
European Commission 2020c. Commission Staff Working Document. Clean Energy Transition – Technologies and Innovations. SWD(2020) 953 final. SWD on the report on clean energy competitiveness | Energy (europa.eu)
European Commission 2020d. Staff Working Document accompanying the EU Strategy to harness the potential of offshore renewable energy for a climate neutral future. SWD(2020) 273 final. Available at: https://ec.europa.eu/energy/sites/ener/files/staff_working_document_on_the_offshore_renewable_energy_strategy.pdf
European Commission 2020e. Commission notice. Guidance document on wind energy developments and EU nature legislation. C(2020) 7730 final. Available at: https://ec.europa.eu/environment/nature/natura2000/management/docs/wind_farms_en.pdf
French Government 2019.Integrated national energy and climate plan for France. Available at: https://ec.europa.eu/energy/sites/ener/files/documents/fr_final_necp_main_en.pdf
German Federal Government 2019. Integrated national energy and climate plan. Available at: https://ec.europa.eu/energy/sites/ener/files/documents/de_final_necp_main_en.pdf
ICES 2019. OSPAR request to advise on the current state and knowledge of studies into the deployment and environmental impacts of wet renewable technologies and marine energy storage systems. In Report of the ICES Advisory Committee, 2019. ICES Advice 2019, sr.2019.05, Available at: https://doi.org/10.17895/ices.advice.4894
Irish Department of Communications, Climate Action and Environment 2019. National energy and climate plan. Available at: https://ec.europa.eu/energy/sites/ener/files/documents/ie_final_necp_main_en.pdf
Netherlands Ministry of Economic Affairs and Climate Policy, 2020. Development framework for offshore wind energy. Development Framework for Offshore Wind Energy (rvo.nl)
Noordzeeloket 2020. Offshore wind ecological programme (WOZEP). Information accessed November 2020. Available at: https://www.noordzeeloket.nl/en/functions-and-use/offshore-wind-energy/ecology/offshore-wind/
Norwegian Ministries 2019. Blue Opportunities - the Norwegian Government's updated ocean strategy. Blue Opportunities (regjeringen.no)
NSEC 2020. The North Seas Energy Cooperation. The North Seas Energy Cooperation | Energy (europa.eu)
OSPAR 2008. OSPAR Guidance on environmental considerations for offshore wind farm development. Agreement 2008-03. Available at: https://www.ospar.org/work-areas/eiha/offshore-renewables
Portuguese Government 2019. National energy and climate plan. Available at: https://ec.europa.eu/energy/sites/ener/files/documents/pt_final_necp_main_en.pdf
Rijkswaterstaat 2020. Application of Ecosystem Services to support decision making in OSPAR activities. A case study of upscaling offshore wind farms in the North Sea region. Available at: https://www.noordzeeloket.nl/publish/pages/182023/application_of_es_to_support_decision-making_within_ospar_final.pdf
Scottish Government 2020a. Offshore wind policy statement. Available at: https://www.gov.scot/publications/offshore-wind-policy-statement
Scottish Government 2020b. Sectoral plan for offshore wind energy. Available at: https://www.gov.scot/publications/sectoral-marine-plan-offshore-wind-energy/
SEV 2020a. Vestmannasund project update. News release of 31/10/2020 (and earlier news releases). Available at www.sev.fo
SEV 2020b. The Faroes’ first offshore wind farm. News release of 17/09/2020. Available at www.sev.fo
Spanish Government 2019. Integrated national energy and climate plan. Available at: https://ec.europa.eu/energy/sites/ener/files/documents/es_final_necp_main_en.pdf
The Crown Estate 2020. The Offshore Wind Evidence and Change Programme. Information accessed November 2020. Available at: https://www.thecrownestate.co.uk/en-gb/what-we-do/on-the-seabed/energy/a-sustainable-future-for-offshore-wind/
UK Government 2020. The ten point plan for a green industrial revolution. Available at: https://www.gov.uk/government/publications/the-ten-point-plan-for-a-green-industrial-revolution/
UK Government (2020b). Energy white paper: Powering our net zero future (updated 18 December 2020). Available at: https://assets.publishing.service.gov.uk/government/uploads/system/uploads/attachment_data/file/945899/201216_BEIS_EWP_Command_Paper_Accessible.pdf
WindEurope 2011. The European offshore wind industry – key trends and statistics 2010. Available at: https://windeurope.org/about-wind/statistics/offshore/key-trends-2010/
WindEurope 2019. Our energy, our future. How offshore wind will help Europe go carbon-neutral. Available at: https://windeurope.org/about-wind/reports/our-energy-our-future/
WindEurope 2020. Offshore wind in Europe – key trends and statistics 2019. Available at: https://windeurope.org/data-and-analysis/product/offshore-wind-in-europe-key-trends-and-statistics-2019/