Concentrations of Dissolved Oxygen Near the Seafloor in the Greater North Sea, Celtic Seas and Bay of Biscay and Iberian Coast
Background
OSPAR’s strategic objective with regard to eutrophication is to tackle eutrophication through limiting inputs of nutrients and organic matter to levels that do not give rise to adverse effects on the marine environment, with the vision of a clean, healthy and biologically diverse North-East Atlantic (OSPAR, 2021). Dissolved oxygen is one of a suite of eutrophication indicators including nutrient inputs and concentrations, chlorophyll and dissolved oxygen. When assessed and considered together, this multi-step method constitutes the OSPAR Common Procedure to diagnose eutrophication (Figure 1).
Excessive enrichment of marine water with nutrients may lead to algal (phytoplankton) blooms, with the possible consequence of undesirable disturbance to the balance of organisms in the marine ecosystem (Figure 2). Undesirable disturbance includes shifts in the composition and extent of flora and fauna, and depletion of oxygen caused by decomposition of accumulated organic material produced by phytoplankton or seaweed communities during their growing seasons. Oxygen depletion may result in behavioural changes or death of fish and bottom fauna and flora. Negative effects on the bottom fauna reduce the amount of food available for fish and hence the productivity of fish populations. Oxygen concentrations above 6mg/l are considered to support marine life with minimal problems, while concentrations less than 2mg/l (hypoxia, i.e., oxygen deficiency) are considered to cause severe problems (Best et al., 2007; Levin et al., 2009). Observations from the Baltic Sea show that when the oxygen content in bottom waters is very low, the only organisms able to thrive are bacteria that live on and in the seafloor.
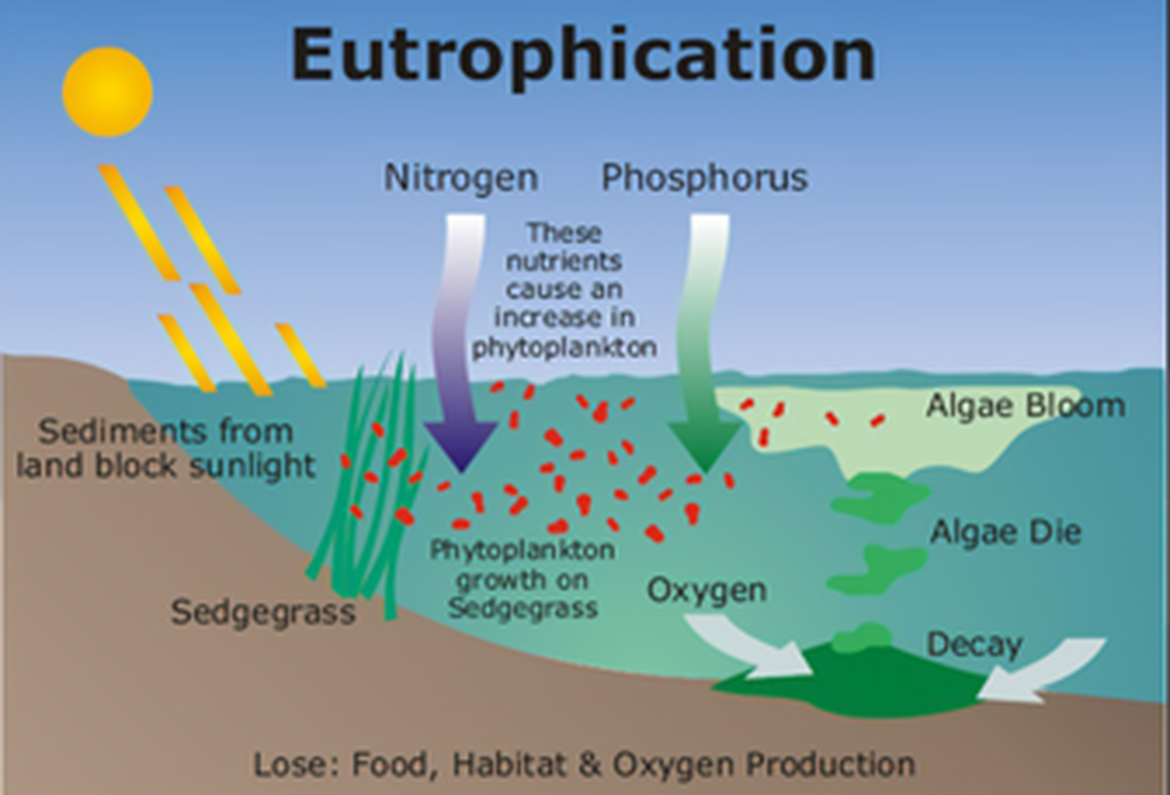
Figure 2: Schematic of eutrophic conditions, dissolved oxygen greatly increases during the day, but is greatly reduced after dark by the respiring algae and by microorganisms that feed on the increasing mass of dead algae. When dissolved oxygen levels decline to hypoxic levels, fish and other marine animals suffocate.
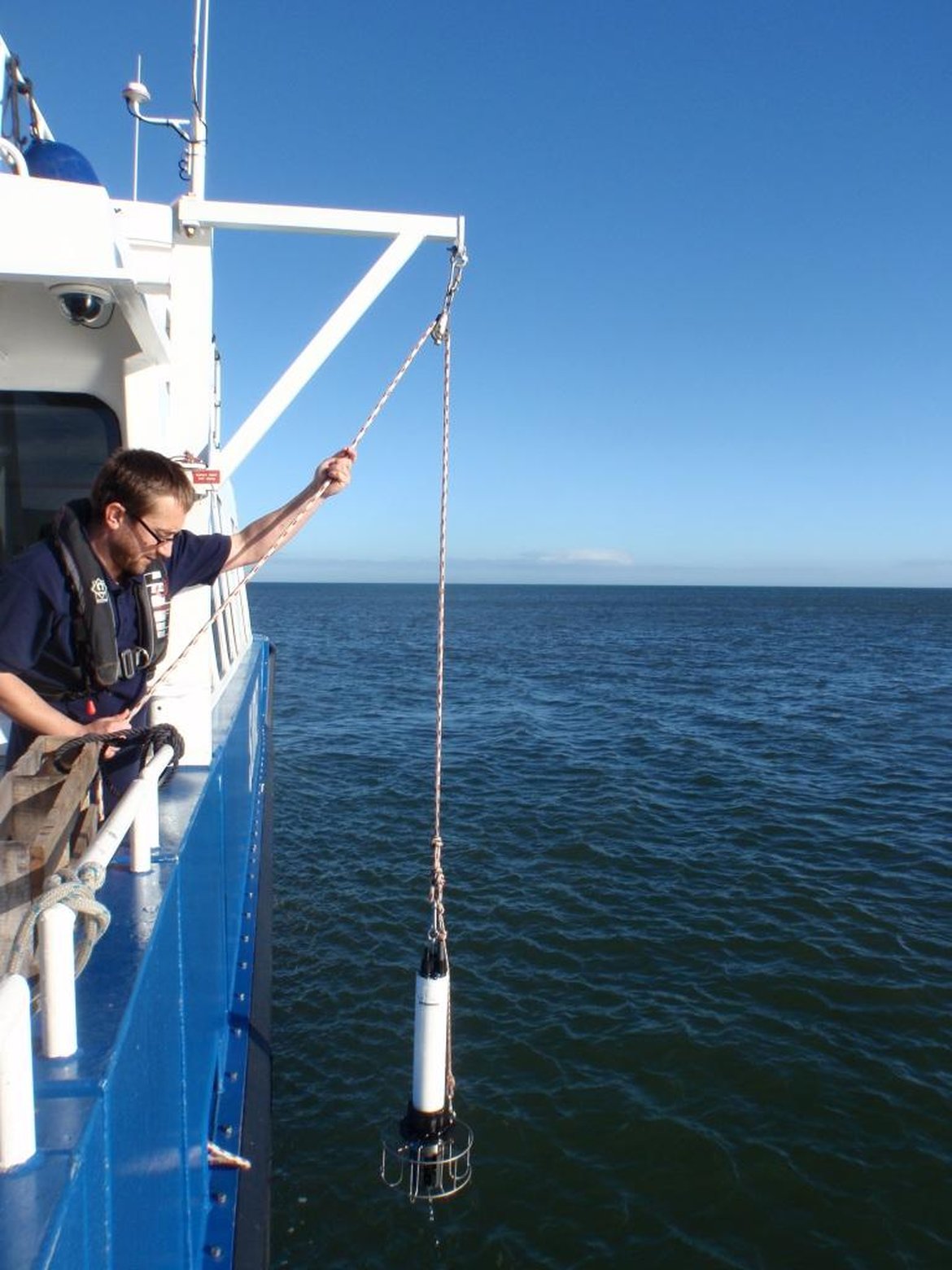
Figure 1: Seawater sampling for dissolved oxygen (© Mike Best, Environment Agency, United Kingdom)
The North-East Atlantic Environment Strategy (NEAES) 2030 contains the OSPAR Commission’s strategic objective to achieve clean seas for the North-East Atlantic by 2030. This includes tackling eutrophication, ‘through limiting inputs of nutrients and organic matter to levels that do not give rise to adverse effects on the marine environment’ (OSPAR, 2021). This strategic objective requires assessment of eutrophication to be based on the ecological consequences of nutrient enrichment and not just on nutrient enrichment alone, i.e., finding reliable evidence for accelerated growth of algae and / or macrophytes caused by anthropogenic nutrient enrichment, leading to undesirable disturbance. Eutrophication is diagnosed using OSPAR’s harmonised criteria of nutrient inputs, concentrations and ratios, chlorophyll concentrations, phytoplankton indicator species, macrophytes, dissolved oxygen levels, incidence of fish kills and changes in zoobenthos (OSPAR, 2022). As there is no single indicator of disturbance caused by marine eutrophication, OSPAR applies a multi-step method using the harmonised criteria.
The concentration of dissolved oxygen was assessed as an indicator of indirect effects of nutrient enrichment and as one of four common indicators. Low levels of oxygen (hypoxia) or no oxygen levels (anoxia) can occur when excess organic materials, such as large algal blooms, are decomposed by microorganisms. During this decomposition process, dissolved oxygen in the water is consumed, resulting in low oxygen levels that can impact on marine organisms. Low oxygen levels often occur in the bottom of the water column and primarily affect organisms that live in the sediments, though these impacts can reverberate through the marine system.
Seasonal oxygen depletion can be a natural localised process, particularly where the water column stratifies, thus isolating water beneath from oxygenated waters above. Although oxygen depletion can be a direct effect of nutrient enrichment, other pressures often complicate the identification of causal links between disturbances and nutrient enrichment. Seasonal oxygen depletion can be a natural localised process, particularly where the water column stratifies seasonally. Temperature, salinity and depth are considered as co-factors that determine the sensitivity of an area to increases in nutrient input, where the natural processes can be intensified by additional nutrient inputs.
Depletion of oxygen is driven by decomposition of accumulated organic material produced by algae (phytoplankton) or seaweed communities. Thus, changes in near-bed oxygen concentration are determined by the quantity of organic matter input to the seafloor, the speed of bacteria decay, and the water renewal rate, hence the nutrient input can be a key factor in driving oxygen reduction. Dissolved oxygen levels fluctuate periodically, seasonally and even as part of the natural daily ecology of the aquatic resource, making it difficult to monitor and measure acute changes.
Declining oxygen (hereafter DO) is a threat to many coastal and marine areas around the world. Hypoxia (dissolved oxygen concentration below 2mg/l) and less severely depleted dissolved oxygen concentrations have the potential to cause lethal and sub-lethal effects to a range of benthic organisms and fish, which may cause loss of biodiversity in these impacted ecosystems. Increasing eutrophication-induced hypoxia has led to dead zones affecting more than 245 000km2 of marine ecosystems worldwide (Diaz and Rosenberg, 2008). It is not always easy to show the full extent of declining oxygen, and modelling can be a useful method to demonstrate where depletion of dissolved oxygen may occur. Metrics such as the Oxygen Deficiency Index (ODI) based on the key parameters of depth, stratification period and net Primary Production can identify areas which are susceptible to dissolved oxygen depletion (Grosse et al., 2016). Shallow stratified areas are most susceptible to oxygen depletion as they have a limited volume of water below the thermocline that can be oxygen depleted within a summer period, before autumn storms break up the thermocline. Areas such as the Eastern North Sea (ENS) are one such area and are susceptible to oxygen depletion (Figure a).
Anthropogenic pressures on water bodies may create new areas of oxygen depletion or exacerbate areas which have a natural tendency for low oxygen concentrations. For example, increased dissolved nutrient inputs have resulted in an anthropogenically induced region of dissolved oxygen below 2ml/l (2,9mg/l) in large areas (70 000km2) of the Baltic Sea (Conley et al., 2002). Large quantities of nutrients have been discharged into the Black Sea with 90% of its volume classified as anoxic (Mee, 1992). New areas of hypoxia are being observed globally (Chan et al., 2008).
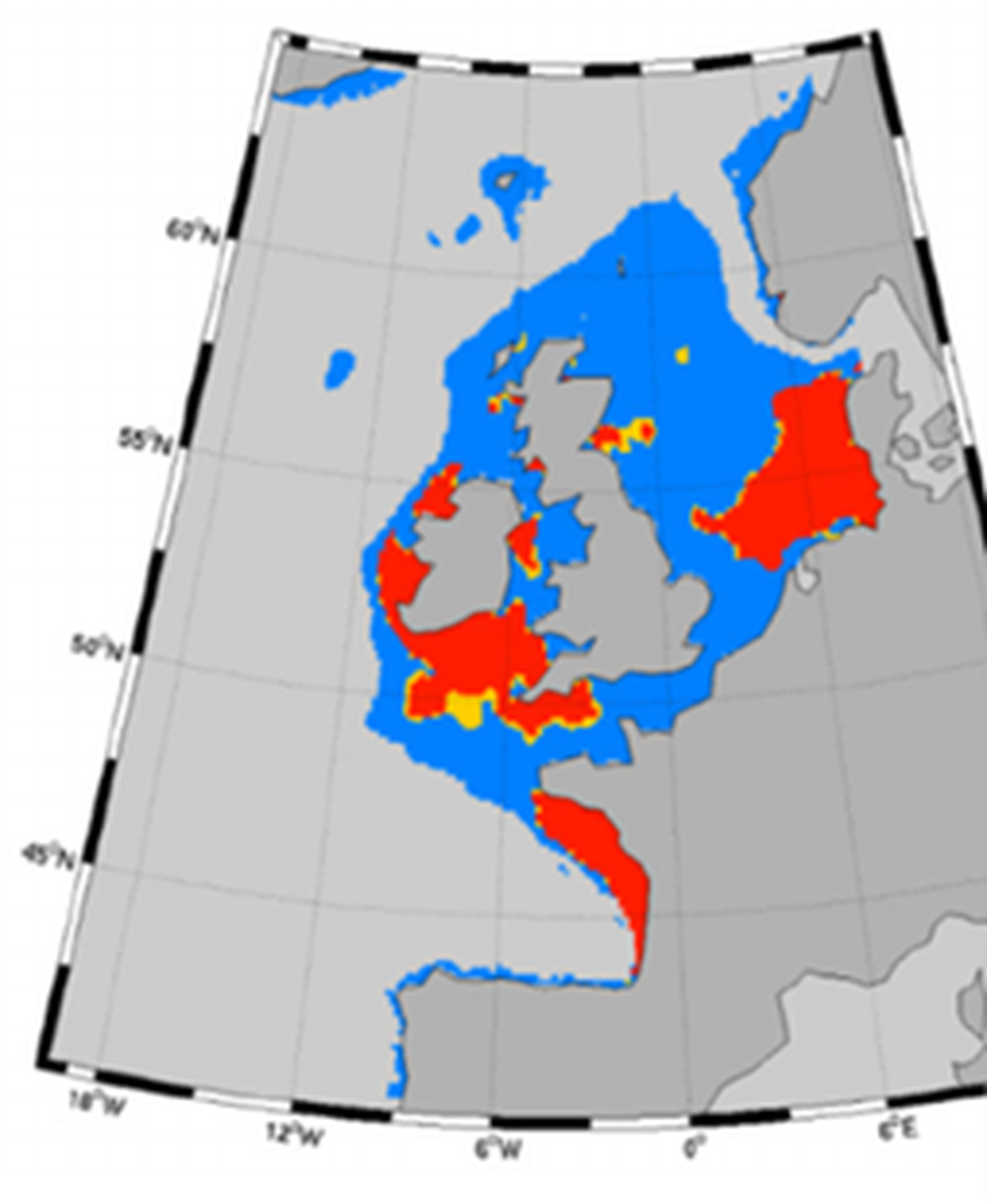
Figure a: Model assessment of areas susceptible to oxygen deficiency with at least one day per year beneath 6mg/l. From Ciavatta 2016.
Nutrient inputs have always been considered a key driver in oxygen deficiency in the North-East Atlantic. Nutrient inputs from adjacent North Sea regions and North-East Atlantic contributed to the evolution of hypoxia in the 1980s (Brockmann and Eberlein, 1986; Brockmann et al., 1988; Artioli et al., 2008; Gröger et al., 2013). Atmospheric N deposition has also been found to be linked to near-bottom O2 concentrations in the south-eastern North Sea. In the North Sea, the hypoxic conditions of the 1980s caused the death of benthic and demersal species (von Westernhagen and Dethlefsen, 1983, Peeters et al., 1995).
The North-East Atlantic ocean is a large, dynamic area, with some areas more susceptible to oxygen deficiency. Große et al., (2016), through the application of a physical-biogeochemical model, related the distinct seasonal thermal stratification in the southern North Seas to the occurrence of thin sub-thermocline layers in the region of intermediate depth, and high local near-surface net primary production (NPP) constituted key factors in that susceptibility. Different studies showed that these occurrences of low DO conditions evolved during seasonal stratification (Peeters et al., 1995), driven by eutrophication due to excess riverine nutrient loads (Brockmann and Eberlein, 1986; Brockmann et al., 1988; Peeters et al., 1995). N sources have a strong influence on the oxygen dynamics in the southern and south-eastern North Sea.
During future climate change, simultaneous shifts in temperature, CO2, and hypoxia levels will enhance sensitivity to environmental extremes relative to a change in just one of these variables. As more carbon dioxide is released enhancing the greenhouse effect, much of the heat is absorbed by the oceans. In turn, this warmer water can hold less oxygen. Scientists estimate that between 1960 and 2010, the amount of the gas dissolved in the oceans declined by 2%. Experimental evidence indicates that as temperatures rise above thermal optima, oxygen threshold (as % saturation) for hypoxia rises as a consequence of the steep increase in respiratory demand. Periods of super saturation can provide additional resources to combat periods of low oxygen and warmer temperatures. Recent work in the Red Sea demonstrates that hyperoxia during the warmest hours of the day enhances the survival scope of marine animals under high to extreme water temperatures (Giomi et al., 2019). The interaction between temperature and oxygen needs to be considered when assessing long-term changes in offshore waters. However, in coastal waters, biological processes tend to be the main control of oxygen concentration, outweighing the decline of oxygen solubility with increasing temperature (Meire et al., 2013) and the different conditions need to be considered when assessing coastal to offshore waters.
Near seafloor oxygen concentrations >6mg/l are considered to cause minimal problems to marine life (Best et al., 2007), while concentrations <2mg/l (hypoxia) are considered to cause severe problems (Levin et al., 2009). Assessment levels used by OSPAR countries applying the Common Procedure (OSPAR 2013-8) ranged from 2 to 6mg/l and were applied over specified assessment periods. For the fourth application of the Common Procedure (OSPAR 2022-07), this period is 2015–2020 and again, the common threshold of 6mg/l is used. The assessment period includes the period between July and October, inclusive, capturing the critical period of high biological growth. The process of assessment is shown in Figure b. Data is filtered first to include only oxygen concentrations close to the seabed, in waters shallower than 500 m as oxygen depletion in deeper waters is unlikely to be due to eutrophication. The assessment process selects the deepest sample at each station and only used data within 10 m of the seabed. Approximately 1/3rd of the data does not have water column depth and this is filled in using bathymetry from EMODNET as a proxy for water depth. Calculation of the 5th percentile is applied at the assessment area scale.
Individual parameters will be assessed against their area-specific assessment levels by calculating an ecological quality ratio. An Ecological Quality Ratio (EQR) for dissolved oxygen is calculated from the measured assessment value (mean 5th percentile DO). Whilst EQRs for nutrients and chlorophyll are obtained by dividing the assessment data by the respective reference concentration, it is the opposite for dissolved oxygen with the reference condition divided by the assessment value. The EQRs are then scaled to a uniform scale (Figure c).
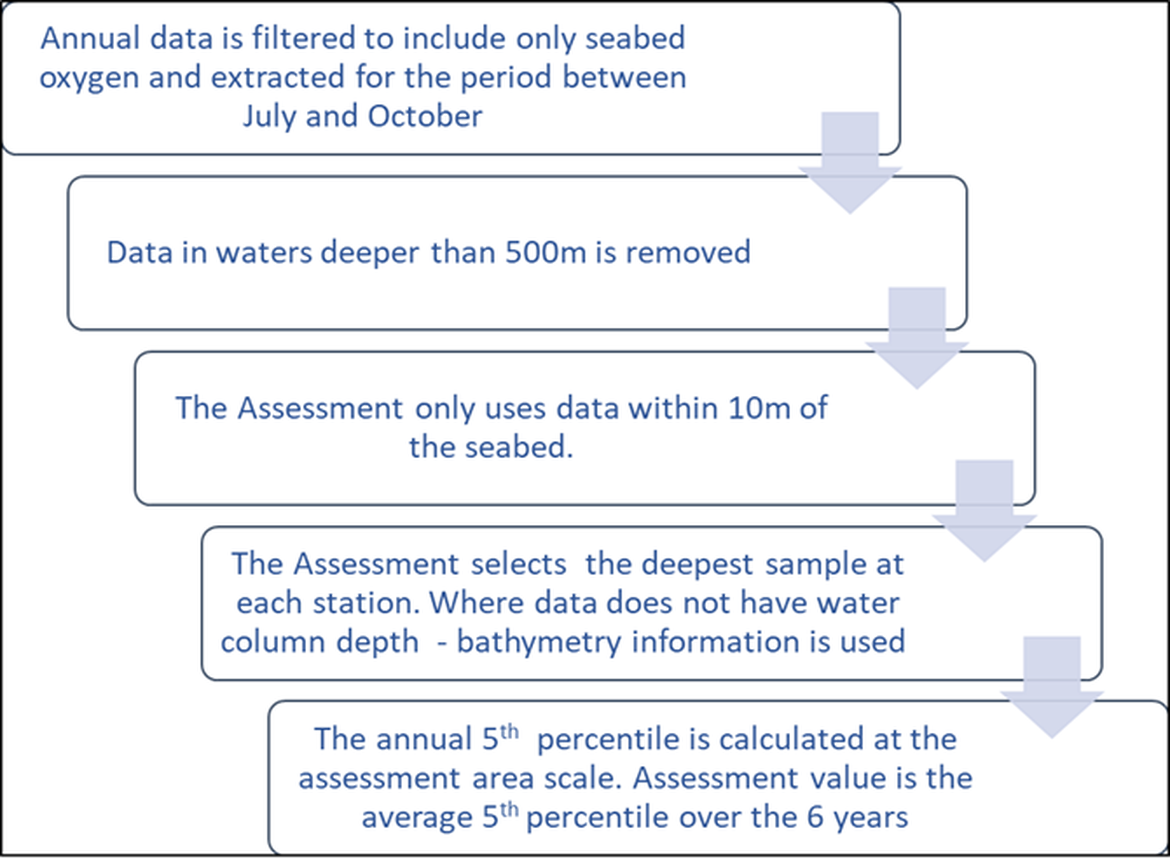
Figure b: Process of selecting dissolved oxygen data from COMPEAT
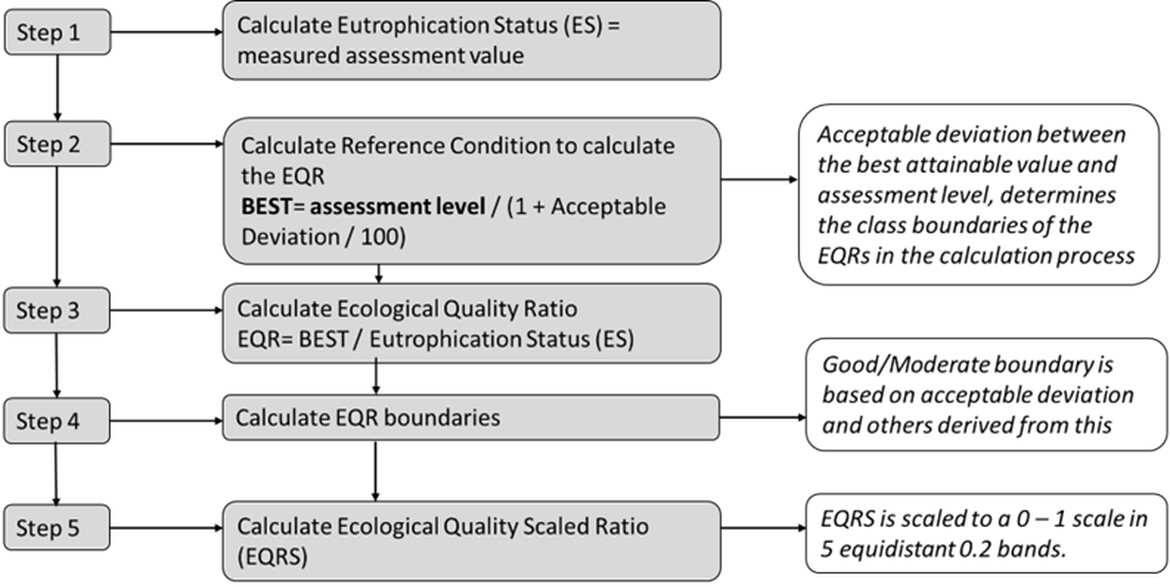
Figure c: Calculation of Ecological Quality Scaled Ratio for the final assessment value
In addition, where there was sufficient data from the period 1990–2020, time series of the assessment statistic for each year were constructed and used to assess the direction of change (increasing or decreasing oxygen) through trend assessments. Data from the period 1990–2020 were used to plot trends to identify areas of concern where oxygen levels are declining, even in non-problem areas where the threshold value was not exceeded.
Additionally, EQRs for finer scale grids within each assessment area were used to look at particular areas in more detail, as part of the supporting data. The fine scale assessment helps identify high risk areas, particularly in the larger assessment areas.
COMP4 Assessment Period
For the period relevant to the fourth application of the Common Procedure (2015–2020), data on oxygen concentrations were analysed by OSPAR assessment areas (Table a). The mean of 5th percentile oxygen concentrations across the 7 years in the assessment calculated as an EQR for each assessment area using five equidistant ranges for EQRS values (0,2, 0,4, 0,6, 0,8) representing bad, poor, moderate, good and high status. These EQR values were summarised in maps for each assessment area.
Data
Data on dissolved oxygen concentrations for the period 1990–2020 and all supporting information (e.g., latitude, longitude, water column depth, sample depth, temperature, salinity) were obtained from ICES (Figure d). Datasets were extracted from COMPEAT (ICES) in June 2022 from 35N to 63N, 13W to 16E (to encompass OSPAR Maritime Area). Data were filtered for the assessment extracting only data from within 10 m of the seafloor in marine waters during the stratification season (1 July–31 October) and then the deepest sample was selected for the analyses. Percentage saturation of dissolved oxygen was calculated from oxygen concentrations, temperature and salinity using the Benson method (Montgomery et al., 1964). Samples taken in water column deeper than 500 m were not included in the analyses. The assessment does not include the coastal waters <1nm as WFD areas not assessed in COMPEAT, but data is in ICES for some Contracting Parties.
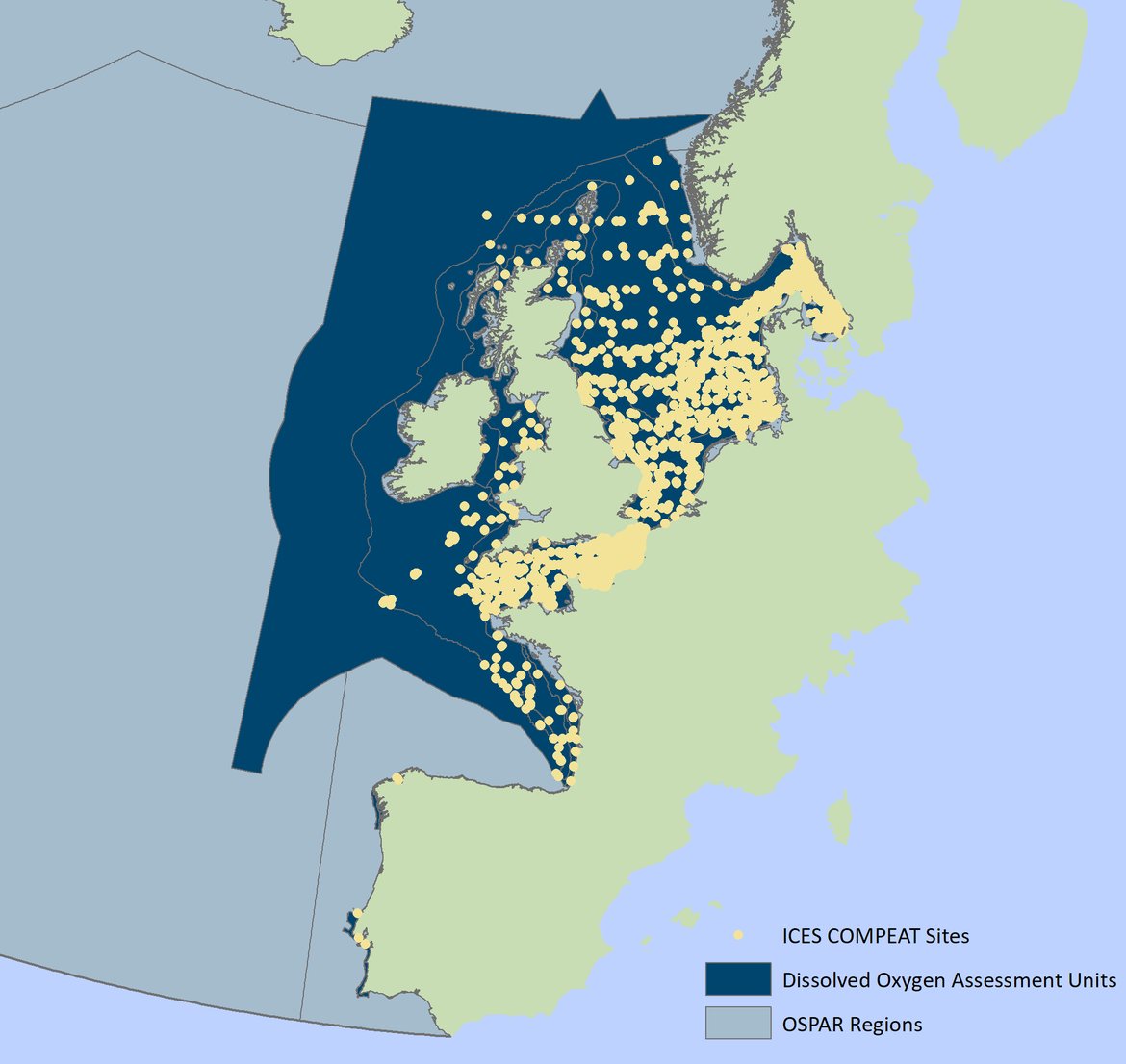
Figure d: Assessed area showing sites where ICES data were available for the period 2015–2020 Filtered by season (stratification season 1 July–31 October), depth (within 10 m of the seafloor) to obtain the specific data required for the assessments
Assessment areas
Two scales of data aggregation were adopted for the assessment, the large-scale common assessment areas, and smaller gridded rectangles.
Dissolved oxygen concentrations were assessed in all COMP4 assessment areas in OSPAR Regions II, III and IV (Greater North Sea, Celtic Seas, Bay of Biscay and Iberian Coast). For the 4th application of the Common Procedure, new harmonised assessment areas have been defined that represent ecologically relevant areas in OSPAR Regions II, III and IV. These assessment areas can be categorised in four different types with distinct characteristics: river plumes, coastal waters, shelf areas, and oceanic areas. River plumes are areas near and downstream from the mouths of large rivers, with generally relatively low and variable salinity and strongly influenced by river discharges. Coastal waters are areas other than river plumes, with average salinity ≤34,5. Shelf areas are areas with average salinity >34,5 and depths <200 m, while oceanic waters are the areas off the continental shelf with a depth of >200 m. The new assessment areas (Figure e) are fully described in OSPAR (2022).
Figure e: Overview of ecologically relevant assessment areas based on duration of stratification, mean surface salinity, depth, suspended particulate matter and primary production. Assessment area codes and names are listed in Table a. Available at: https://odims.ospar.org/en/submissions/ospar_comp_au_2023_01/
Categories | Area code | Area name | Salinity surface mean | Depth mean | OSPAR Region |
---|---|---|---|---|---|
River plumes assessment units | ADPM | Adour plume | 34.4 | 87 | IV |
ELPM | Elbe plume | 30.8 | 18 | II | |
EMPM | Ems plume | 31.4 | 19 | II | |
GDPM | Gironde plume | 33.5 | 34 | IV | |
HPM | Humber plume | 33.5 | 16 | II | |
LBPM | Liverpool Bay plume | 30.6 | 15 | III | |
LPM | Loire plume | 33.8 | 38 | IV | |
MPM | Meuse plume | 29.3 | 16 | II | |
RHPM | Rhine plume | 31.0 | 17 | II | |
SCHPM1 | Scheldt plume 1 | 31.4 | 13 | II | |
SCHPM2 | Scheldt plume 2 | 30.9 | 15 | II | |
SHPM | Shannon plume | 34.1 | 61 | III | |
SPM | Seine plume | 31.8 | 25 | II | |
THPM | Thames plume | 34.4 | 22 | II | |
Coastal assessment units | CFR | Coastal FR channel | 34.2 | 33 | II |
CIRL | Coastal IRL 3 | 34.0 | 65 | III | |
CNOR1 | Coastal NOR 1 | 34.3 | 190 | II | |
CNOR2 | Coastal NOR 2 | 34.0 | 217 | II | |
CNOR3 | Coastal NOR 3 | 32.4 | 171 | II | |
CUK1 | Coastal UK 1 | 34.5 | 60 | III | |
CUKC | Coastal UK channel | 34.8 | 37 | II | |
CWAC | Coastal Waters AC | - | - | IV | |
CWBC | Coastal Waters BC | - | - | IV | |
CWCC | Coastal Waters CC | - | - | IV | |
ECPM1 | East Coast (permanently mixed) 1 | 34.8 | 73 | II | |
ECPM2 | East Coast (permanently mixed) 2 | 34.5 | 43 | II | |
GBC | German Bight central | 33.4 | 39 | II | |
IRS | Irish Sea | 33.7 | 65 | III | |
KC | Kattegat Coastal | 25.7 | 21 | II | |
KD | Kattegat Deep | 27.6 | 50 | II | |
NAAC1A | Noratlantic Area NOR-NorC1 | - | - | IV | |
NAAC1B | Noratlantic Area NOR-NorC1 | - | - | IV | |
NAAC1C | Noratlantic Area NOR-NorC1 | - | - | IV | |
NAAC1D | Noratlantic Area NOR-NorC1 | - | - | IV | |
NAAC2 | Noratlantic Area NOR-NorC2 | - | - | IV | |
NAAC3 | Noratlantic Area NOR-NorC3 | - | - | IV | |
OC | Outer Coastal DEDK | - | - | II | |
SAAC1 | Sudatlantic Area SUD-C1 | - | - | IV | |
SAAC2 | Sudatlantic Area SUD-C2 | - | - | IV | |
SAAP2 | Sudatlantic Area SUD-P2 | - | - | IV | |
SNS | Southern North Sea | 34.3 | 32 | II | |
Shelf assessment units | ASS | Atlantic Seasonally Stratified | 35.2 | 134 | III, IV |
CCTI | Channel coastal shelf tidal influenced | 34.8 | 40 | II | |
CWM | Channel well mixed | 35.1 | 77 | II, III | |
CWMTI | Channel well mixed tidal influenced | 35.0 | 59 | II | |
DB | Dogger Bank | 35.1 | 28 | II | |
ENS | Eastern North Sea | 34.8 | 43 | II | |
GBCW | Gulf of Biscay coastal waters | 34.6 | 53 | IV | |
GBSW | Gulf of Biscay shelf waters | 34.9 | 107 | IV | |
IS1 | Intermittently Stratified 1 | 35.3 | 138 | II, III | |
IS2 | Intermittently Stratified 2 | 35.1 | 102 | II | |
NAAP2 | Noratlantic Area NOR-NorP2 | - | - | IV | |
NAAPF | Noratlantic Area NOR-Plataforma | - | - | IV | |
NNS | Northern North Sea | 35.0 | 121 | II | |
NT | Norwegian Trench | 34.1 | 349 | II | |
SAAP1 | Sudatlantic Area SUD-P1 | - | - | IV | |
SK | Skagerrak | 31.8 | 134 | II | |
SS | Scottish Sea | 35.1 | 89 | II, III | |
Oceanic assessment units | ATL | Atlantic | 35.3 | 2291 | II, IV, V |
NAAO1 | Noratlantic Area NOR-NorO1 | - | - | IV | |
OWAO | Ocean Waters AO | - | - | IV | |
OWBO | Ocean Waters BO | - | - | IV | |
OWCO | Ocean Waters CO | - | - | IV | |
SAAOC | Sudatlantic Area SUD-OCEAN | - | - | IV |
Within each assessment area, smaller scale grids (10, 30, or 60km depending on the size of the assessment area) help to identify localised areas that may be characterised by low oxygen levels (‘hotspots’). The DO assessment was applied to each grid section using data collected between July and October calculating the 5th percentile of the deepest sample within 10m of the seabed. Mean EQR values were calculated for each year, then averaged over the assessment period for a final EQR value for each grid square. The outcome per area was summarised in a map showing the mean EQR value calculated from an average of the annual EQR values.
Trend Assessments
For the full-time series (1990–2020), means of the 5th percentile of the data per stratification season per year were used to identify trends in the data. Trends were assessed using the Mann-Kendall non-parametric test for trend analysis (Mann, 1945; Kendall, 1975). The R library package ‘emon’ was used (Barry and Maxwell, 2015). Where p<0,05, it was assumed that there was a significant trend. Where p>0,05, a trend could not be detected statistically.
Trend assessment was carried out for both dissolved oxygen concentrations and dissolved oxygen saturation. Number of data points available for DO concentrations after application of filters is summarised for the four assessment periods in Table b.
Description | 1990-2000 | 2001-2006 | 2006-2014 | 2015-2020 |
---|---|---|---|---|
Adour plume | 1 | |||
Atlantic Seasonally Stratified | 56 | 8 | 68 | 170 |
Atlantic | 273 | 20 | 28 | 26 |
Channel coastal shelf tidal influenced | 2 | 3 | 67 | |
Coastal FR channel | 2 | 1 | 161 | |
Coastal IRL 3 | 9 | 1 | ||
Coastal NOR 1 | 1 | |||
Coastal NOR 2 | 1 | 4 | ||
Coastal NOR 3 | 1 | 8 | 1 | 4 |
Outer Coastal DEDK | 464 | 259 | 215 | 340 |
Coastal UK 1 | 3 | 11 | 11 | |
Coastal UK channel | 5 | 34 | ||
Coastal Waters BC (D5) | 1 | 3 | ||
Channel well mixed | 6 | 6 | 13 | 141 |
Channel well mixed tidal influenced | 6 | 4 | 134 | |
Dogger Bank | 29 | 101 | 33 | 68 |
East Coast (permanently mixed) 2 | 1 | |||
Elbe plume | 455 | 272 | 213 | 149 |
Ems plume | 33 | 47 | 84 | 65 |
Eastern North Sea | 211 | 323 | 329 | 678 |
German Bight central | 83 | 121 | 125 | 233 |
Gulf of Biscay coastal waters | 11 | |||
Gulf of Biscay shelf waters | 20 | |||
Gironde plume | 2 | |||
Humber plume | 5 | |||
Irish Sea | 23 | 19 | 17 | |
Intermittently Stratified 1 | 41 | 6 | 20 | 26 |
Intermittently Stratified 2 | 2 | 18 | 14 | 35 |
Kattegat Coastal | 730 | 405 | 517 | 440 |
Kattegat Deep | 524 | 314 | 372 | 324 |
Liverpool Bay plume | 1 | 1 | 1 | |
Meuse plume | 4 | 31 | 34 | 25 |
Noratlantic Area NOR-NorC2(D5) | 7 | 23 | ||
Noratlantic Area NOR-NorC3(D5) | 34 | 12 | ||
Noratlantic Area NOR-NorO1(D5) | 2 | |||
Noratlantic Area NOR-NorP2(D5) | 27 | |||
Noratlantic Area NOR-Plataforma | 23 | |||
Northern North Sea | 356 | 743 | 449 | 125 |
Norwegian Trench | 78 | 54 | 74 | 102 |
Ocean Waters AO (D5) | 4 | 1 | ||
Ocean Waters CO (D5) | 2 | 3 | ||
Rhine plume | 27 | 17 | 37 | 23 |
Sudatlantic Area SUD-C1(D5) | 4 | 1 | ||
Sudatlantic Area SUD-P2(D5) | 2 | |||
Scheldt plume 1 | 23 | 15 | 32 | 32 |
Scheldt plume 2 | 4 | 3 | ||
Skagerak | 203 | 147 | 167 | 193 |
Southern North Sea | 84 | 201 | 233 | 489 |
Seine plume | 13 | 1 | 34 | |
Scottish Sea | 7 | 26 | 56 | 98 |
Thames plume | 4 | 26 | 22 |
Results
All but seven assessment areas have been classified as good or high status, based on the mean 5th percentile value of DO being greater or equal to a threshold value of 6,0mg/l for the period 2015-2020. There are seven (7) assessment areas which have mean 5th percentile DO values falling below 6,0mg/l as follows: Adour Plume (4,4mg/l), Kattegat Coastal (3,92mg/l), Kattegat Deep (4,70mg/l), Eastern North Sea (5,69mg/l), Gironde Plume (5,3mg/l), Gulf of Biscay coastal (5,56mg/l) and Gulf of Biscay shelf waters (5,86mg/l) (Figure 3).
Of the seven assessment areas that fall below the good/moderate threshold, the Mann Kendall trend analysis does not show any statistically significant trends, with the exception of the Kattegat Coastal region showing an increasing trend (p<0,05) as does Kattegat deep, although it is not statistically significant (p<0,1). However, in areas which have been classified as good or high, five assessment areas showed statistically significant decreasing oxygen concentrations. These include the Atlantic Region (ATL) Atlantic Seasonally Stratified (ASS), Meuse Plume (MPM), Norwegian Trench (NT) and Scheldt Plume (SCHPM1).
The use of saturation, rather than concentration, compensates for trends in oxygen concentration due to temperature. When correcting for temperatures, the Meuse Plume (MPM), Atlantic Region (ATL), Norwegian Trench (NT) and Scheldt Plume (SCHPM1) all still show a statistically significant negative trend. In addition, the Northern North Sea (NNS) also shows a negative trend in decreasing oxygen.
Figure 3: Outcomes of the assessment for dissolved oxygen for the COMP4. Available at: https://odims.ospar.org/en/submissions/ospar_dissolved_oxygen_eqrs_2020_06/
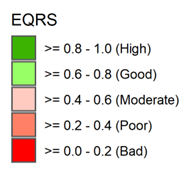
The outcomes of the DO common indicator assessment are presented alongside the calculation of confidence for each assessment area (Figure f). The outcomes are also summarised in greater detail in Table c, with the EQR values presented for each assessment area.
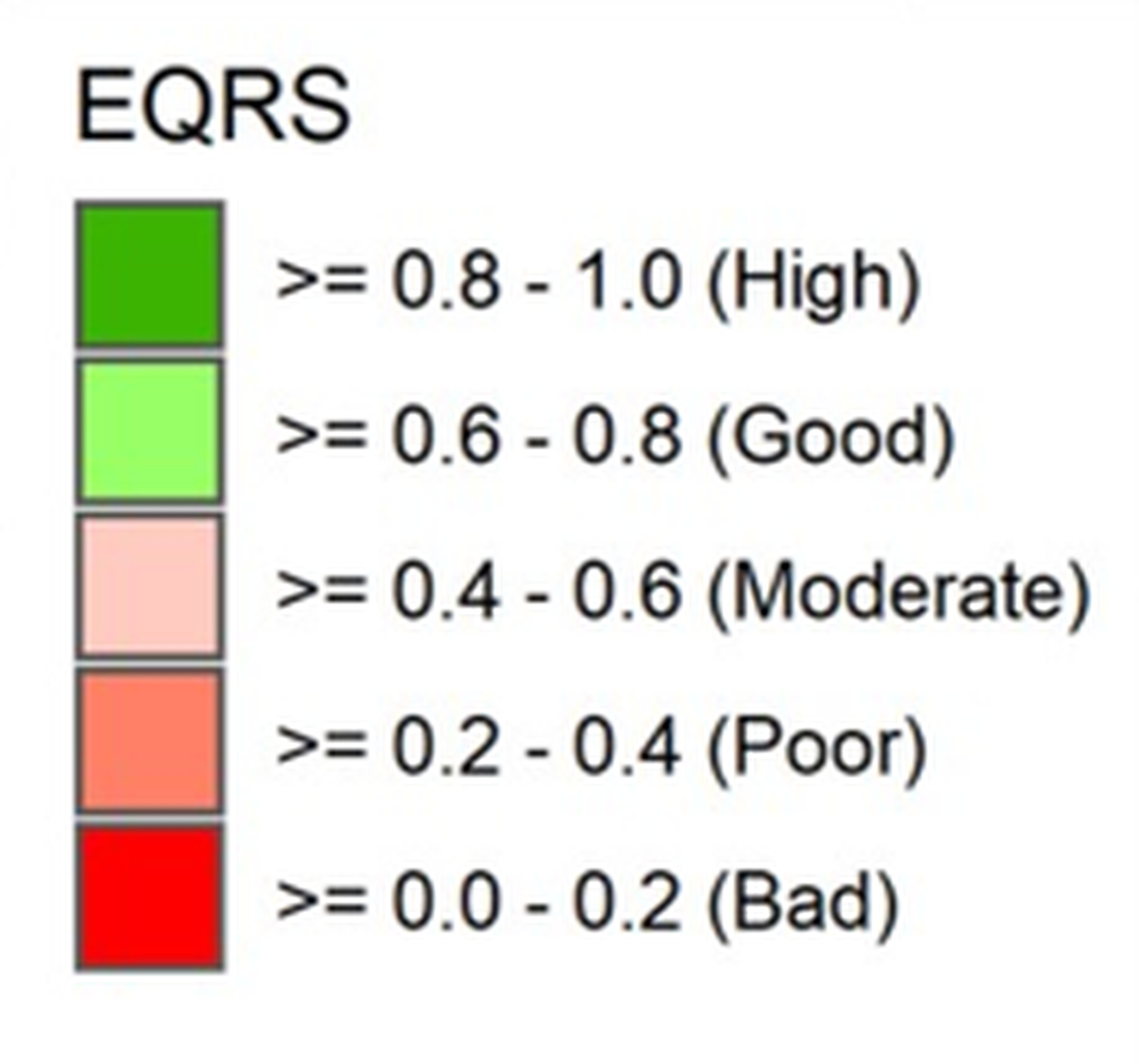
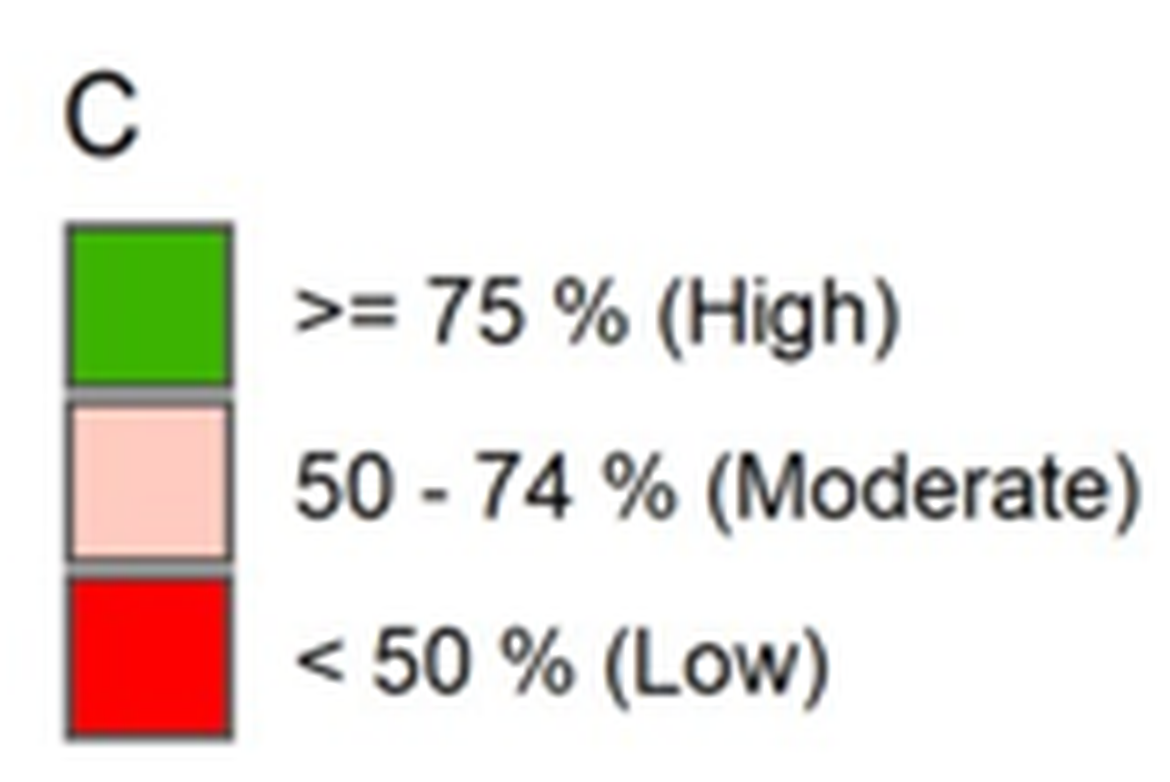
Figure f: Outcomes of the assessment and calculation of confidence for dissolved oxygen for the COMP4
Assessment areas with values below the good/moderate threshold are highlighted in light red (Table c). The summary table also includes the results of the Mann Kendall analysis for the entire period 1990 – 2020. The last column is the p Value and it is highlighted in dark red if it is statistically significant. The sign of MK stat gives the direction of change, with a negative value (-ve) describing a decrease in DO concentrations. Seven assessment areas have been classified as not achieving good status, based on the mean 5th percentile value of DO being less than a threshold value of 6,0mg/l for the period 2015-2020. There are seven assessment areas which have mean 5th percentile DO values falling below 6,0mg/L as follows: Adour Plume (4,4mg/l), Kattegat Coastal (3,92mg/l), Kattegat Deep (4,70mg/l), Eastern North Sea (5,69mg/l), Gironde Plume (5,3mg/l), Gulf of Biscay coastal (5,56mg/l) and Gulf of Biscay shelf waters (5,86mg/l). All other assessment areas achieved good or high status. However, alongside the seven assessment areas which have not achieved good status, there are also five assessment areas which have statistically significant decreasing oxygen concentration in the Atlantic Region (ATL) Atlantic Seasonally Stratified (ASS), Meuse Plume (MPM), Norwegian Trench (NT), Scheldt Plume (SCH1) (Table c).
Table c: For each Area, the final value for the 5th percentile DO value and the calculated EQR where assessment areas falling below the good/moderate boundary (0,6) are highlighted in pink. The Mann Kendall (MK) statistics including (No yrs, MK stat and P value are also provided to indicate trend. A -ve MK stat shows a decreasing trend of oxygen concentration, with p values < 0,05 showing statistically significant trend and highlighted in red).
Code | Assessment area | 5th %ile | NO | EQR | No yrs | Mk stat | P val |
---|---|---|---|---|---|---|---|
ADPM | Adour Plume | 4,44 | 1 | 0,44 | 1 | 0 | 1 |
ATL | Atlantic | 6,64 | 26 | 0,66 | 22 | -115 | <,01 |
ASS | Atlantic Seasonally Stratified | 6,29 | 170 | 0,63 | 18 | -63 | 0,02 |
CCTI | Channel coastal shelf tidal influenced | 9,73 | 67 | 0,96 | 8 | 14 | 0,1 |
CWM | Channel well mixed | 7,99 | 141 | 0,79 | 10 | 6 | 0,66 |
CWMTI | Channel well mixed tidal influenced | 9,31 | 134 | 0,93 | 8 | 16 | 0,06 |
CFR | Coastal FR channel | 9,62 | 161 | 0,95 | 7 | 9 | 0,25 |
CIRL | Coastal IRL 3 | 6,6 | 1 | 0,66 | 2 | -1 | 1 |
CNOR1 | Coastal NOR 1 | 1 | 0 | 1 | |||
CNOR2 | Coastal NOR 2 | 3 | -1 | 1 | |||
CNOR3 | Coastal NOR 3 | 8,14 | 4 | 0,81 | 6 | 7 | 0,28 |
CUK1 | Coastal UK 1 | 7,9 | 11 | 0,79 | 6 | 1 | 1 |
CUKC | Coastal UK channel | 9,11 | 34 | 0,9 | 6 | -5 | 0,47 |
CWBC | Coastal Waters BC | 8,78 | 3 | 0,88 | 2 | 1 | 1 |
DB | Dogger Bank | 7,53 | 68 | 0,75 | 27 | -70 | 0,14 |
ECPM2 | East Coast (permanently mixed) 2 | 7,79 | 1 | 0,78 | 1 | 0 | 1 |
ENS | Eastern North Sea | 5,69 | 678 | 0,57 | 30 | -30 | 0,6 |
ELPM | Elbe Plume | 6,93 | 149 | 0,69 | 32 | 69 | 0,26 |
EMPM | Ems Plume | 6,84 | 65 | 0,68 | 25 | 35 | 0,41 |
GBC | German Bight central | 6,54 | 233 | 0,65 | 29 | 6 | 0,91 |
GDPM | Gironde Plume | 5,3 | 2 | 0,53 | 2 | 1 | 1 |
GBCW | Gulf of Biscay coastal waters | 5,56 | 11 | 0,56 | 3 | 3 | 0,34 |
GBSW | Gulf of Biscay shelf waters | 5,86 | 20 | 0,59 | 5 | 0 | 1 |
HPM | Humber Plume | 8,25 | 5 | 0,83 | 2 | -1 | 1 |
IS1 | Intermittently Stratified 1 | 7,12 | 26 | 0,71 | 16 | -15 | 0,51 |
IS2 | Intermittently Stratified 2 | 7,73 | 35 | 0,77 | 18 | -5 | 0,85 |
IRS | Irish Sea | 7,52 | 17 | 0,75 | 10 | 3 | 0,86 |
KC | Kattegat Coastal | 3,92 | 440 | 0,39 | 32 | 125 | 0,04 |
KD | Kattegat Deep | 4,7 | 324 | 0,47 | 32 | 113 | 0,07 |
LBPM | Liverpool Bay Plume | 8,37 | 1 | 0,84 | 3 | 2 | 0,33 |
MPM | Meuse Plume | 6,23 | 25 | 0,62 | 22 | -116 | 0 |
NAAC2 | Noratlantic Area NOR-NorC2 | 7 | 23 | 0,7 | 7 | 13 | 0,07 |
NAAC3 | Noratlantic Area NOR-NorC3 | 6,69 | 12 | 0,67 | 7 | 7 | 0,38 |
NAAO1 | Noratlantic Area NOR-NorO1 | 1 | 0 | 1 | |||
NAAP2 | Noratlantic Area NOR-NorP2 | 4 | -4 | 0,33 | |||
NAAPF | Noratlantic Area NOR-Plataforma | 5 | -2 | 0,82 | |||
NNS | Northern North Sea | 6,55 | 1252 | 0,66 | 32 | -18 | 0,77 |
NT | Norwegian Trench | 7,68 | 102 | 0,77 | 32 | -131 | 0,03 |
OWAO | Ocean Waters AO | 2 | -1 | 1 | |||
OWCO | Ocean Waters CO | 2 | -1 | 1 | |||
CODEDK | Outer Coastal DEDK | 6,11 | 340 | 0,61 | 31 | -52 | 0,39 |
RHPM | Rhine Plume | 7,43 | 23 | 0,74 | 20 | -39 | 0,21 |
SCHPM1 | Scheldt Plume 1 | 6,55 | 32 | 0,66 | 22 | -81 | 0,03 |
SCHPM2 | Scheldt Plume 2 | 6,74 | 3 | 0,67 | 4 | -2 | 0,75 |
SS | Scottish Sea | 7,51 | 98 | 0,75 | 23 | -15 | 0,69 |
SPM | Seine Plume | 9,66 | 34 | 0,96 | 11 | -2 | 0,88 |
SK | Skagerrak | 6,82 | 193 | 0,68 | 32 | -11 | 0,86 |
SNS | Southern North Sea | 7,08 | 489 | 0,71 | 29 | -79 | 0,14 |
SAAC1 | Sudatlantic Area SUD-C1 | 1 | 0 | 1 | |||
SAAOC | Sudatlantic Area SUD-OCEAN | 1 | 0 | 1 | |||
SAAP2 | Sudatlantic Area SUD-P2 | 1 | 0 | 1 | |||
THPM | Thames Plume | 7,52 | 22 | 0,75 | 11 | -24 | 0,07 |
Three assessment areas (with long term data) that did not achieve good status are Eastern North Sea (ENS), Kattegat Coastal (KC) and Kattegat Deep (KD). The assessment values, calculated from the assessment period (2015–2020) all fall below the good/moderate boundary (EQR = 0,6). Eastern North Sea has a measured assessment value (5th percentile DO) of 5,69mg/L resulting in an EQR of 0,565, calculated from 678 samples, with moderate to high confidence across the range of measures of classification. Kattegat Coastal has a measured assessment value (5th percentile DO) of 3,92mg/L resulting in an EQR of 0,363, calculated from 440 samples, with high confidence of classification across the range of measures of classification. Kattegat Deep has a measured assessment value (5th percentile DO) of 4,70mg/L resulting in an EQR of 0,452, calculated from 324 samples, with a high confidence of classification across the range of measures of classification.
Measured assessment values and EQRS values over the four assessment periods (1990-2000, 2001-2006, 2006–2014, 2015-2020) for Kattegat Deep and Kattegat Coastal show low DO values and EQR values below the good/moderate boundary for all periods (Figure g). For the Eastern North Sea, the measured assessment values for all years fall below the 6mg/L threshold (Figure g), with EQR values less than the 0,6 good/moderate boundary (Figure g).
Figure g: Measured assessment values and EQRS values over the four COMP periods for Eastern North Sea (ENS), Kattegat Coastal (KC) , Kattegat Deep (KD)
The other assessment areas which do not achieve good status are Gironde Plume (GDPM), Adour Plume (ADPM), Gulf of Biscay Coastal Water (GBCW), Gulf of Biscay Shelf Waters (GBSW). The assessment values, calculated from the assessment period (2015–2020) all fall below the good/moderate boundary (0,6).
Gironde Plume has a measured assessment value (5th percentile DO) of 5,3mg/l, resulting in an EQRS of 0,520, calculated from only 2 samples, resulting in a low confidence of classification. The low number of samples (N=2) are not sufficient to assess the area. Gulf of Biscay Coastal Waters has a measured assessment value (5th percentile DO) of 5,56mg/l, resulting in an EQRS of 0,550, calculated from 11 samples. The measured value is close to the good/moderate boundary, resulting in low confidence of classification. Gulf of Biscay shelf waters has a measured assessment value (5th percentile DO) of 5,86mg/l, resulting in an EQRS of 0,584, calculated from 20 samples. The measured value is close to the good/moderate boundary, resulting in low confidence of classification. Whilst there is not enough long-term data to look at the four previous COMP periods, Figure h shows the 5th percentile for Gulf of Biscay shelf and coastal waters and Gironde Plume.
Figure h: Annual values of three assessment areas which have fallen below the Good/Moderate boundary
Adour Plume has a measured assessment value (5th percentile DO) of 4,44mg/l, resulting in an EQRS of 0,422, calculated from only 1 sample, resulting in a low confidence of classification. The low number of samples (N=1) are not sufficient to assess the area
Trend Assessments
Time series are only shown for the regions that fall below the good/moderate boundary (Figure i). The Eastern North Sea, Kattegat Coastal, Kattegat Deep have long-term data available from 1990, demonstrating persistent low dissolved oxygen values but with both Kattegat Coastal and Kattegat Deep showing an increasing trend over the entire period from 1990 to 2020. Of the seven assessment areas that fall below the good/moderate threshold, the Mann Kendall trend analysis does not show any statistically significant trends, with the exception of the Kattegat Coastal region showing an increasing trend (p<0,05) as does Kattegat Deep, although it is not statistically significant (p<0,1). However, the positive development is absent for the last decade, which corresponds with similar results for the other indicators. Eastern North Sea also suggests some improvement in recent years, but this is not significant and will require further observations to resolve the direction of trend (Figure i).
Figure i: Long-term trend in dissolved oxygen concentrations for Eastern North Sea, Kattegat Deep and Kattegat Coastal
The other assessment areas which fall below the good/moderate boundary are Gironde Plume, Adour Plume, Gulf Biscay Coastal Water and Gulf of Biscay shelf waters. However, long-term data for all these areas is limited, making it difficult to draw any conclusions on long-term trends (Figure j). With a low number of samples, and limited long-term data, confidence in the moderate assessment for these four assessment areas is low. Further monitoring and modelling of these areas would be critical to resolve the scale of the issues, and the direction of trend for bottom DO in these four areas.
Figure j: Long-term trend in dissolved oxygen concentrations for Gironde Plume, Adour Plume, Gulf Biscay Coastal Water, Gulf of Biscay shelf waters.
Regions with statistically significant negative trends
Regions which show a statistically significant negative trend include the Atlantic Seasonally Stratified (ASS), Atlantic (ATL), Meuse Plume (MPM), Norwegian Trench (NT), Scheldt Plume 1 (SCHPM1). Whilst they are all classified as good or high status, all these regions demonstrated negative trends in the annual 5th percentile which are statistically significant (p<0.05) over the time period (Figure k). The Kattegat Deep and Kattegat Coastal have increasing trends which are significant at 90% and 95%, respectively (Table c).
Data coverage for Atlantic Seasonally Stratified (ASS) and Atlantic (ATL) is spatially limited resulting in low spatial confidence for these two areas and reducing confidence in the overall trend assessment (Figure k). However, the 5th percentile indicator does reduce in the last two assessment periods as compared to the first two assessment periods, though this may be related to the limited data in these large offshore areas (Figure k). Assessment values and EQRs also decline for Meuse, Norwegian Trench and Scheldt Plume 1 (Figure l).
Figure k: Long-term trend in oxygen concentrations for Atlantic Seasonally Stratified (ASS), Atlantic (ATL), Meuse Plume (MPM), Norwegian Trench (NT), Scheldt Plume 1 (SchPM1). All these areas demonstrate a statistically significant trend.
Figure l: Measured assessment values and EQRS for Atlantic Seasonally Stratified and Atlantic
Figure m: Measured assessment values and EQRS for Meuse Plume, Norwegian Trench and Scheldt Plume 1 over the four assessment periods
Oxygen Saturation
For some species oxygen saturation is a limit on the respiration rate as it controls chemical exchange, e.g., uptake across gills. Trends in oxygen saturation, have therefore been considered in addition to concentration. Saturation has the benefit of compensating for trends in solubility of oxygen due to temperature.
Saturation was calculated using in-situ concentrations of dissolved oxygen, temperature, salinity and pressure. When observing trends in oxygen saturation, which take account of the effect of temperature and salinity on oxygen solubility, the Meuse Plume, Atlantic, Norwegian Trench and Scheldt Plume 1 still all show a statistically significant negative trend, however the Northern North Sea (NNS) also now shows a negative trend. In conjunction with the trends in concentration, these trend results (Table d) do enable an assessment of the components due to warming to be identified. Only in the Atlantic Seasonally Stratified region (ASS) was the decrease in solubility due to temperature the dominant factor in decreased concentration. For the Northern North Sea, the opposite is true, the rate of decrease of saturation is greater than that of concentration. The MK stat for the Meuse Plume is similar for both methods, indicating that temperature effects are not important in this trend.
The Kattegat Coastal region again has a positive trend, demonstrating observable improvements in bottom DO. The nearby areas of Kattegat Deep and Eastern North Sea, whilst falling below moderate in the DO assessment, do not show a statistically significant trend.
Table d: Regions with a statistically significant trend when using saturation (bold at 95%). All trends are decreasing with exception of Kattegat Coastal and Channel well mixed that demonstrate an increasing trend
Code | Description | No_Years | Mk stat | P value |
---|---|---|---|---|
MPM | Meuse Plume | 21 | -130 | 1,00E-04 |
ATL | Atlantic | 21 | -102 | 0,0015 |
NT | Norwegian Trench | 30 | -111 | 0,048 |
SCHPM1 | Scheldt Plume 1 | 22 | -87 | 0,016 |
NNS | Northern North Sea | 31 | -135 | 0,023 |
KC | Kattegat Coastal | 31 | 129 | 0,029 |
SK | Skagerrak | 31 | -115 | 0,053 |
CWM | Channel well mixed | 9 | 18 | 0,074 |
EMPM | Ems plume | 23 | 65 | 0,092 |
The measured assessment values and EQRS values over the assessment periods show a slight improvement on the measured value for Northern North Sea, Skagerrak and Ems Plume, though measured assessment values remain close to the threshold of 6mg/l (Figure n).
Figure n: Measured assessment values and EQRS values for Northern North Sea, Skagerrak and Ems plume over the four COMP periods
Confidence
There are three types of confidence calculated for the DO assessment, they include spatial, temporal and accuracy, which are combined to the overall confidence. The aspect of temporal coverage of monitoring data considers the confidence of the parameter in terms of its year-to-year variation and the continuity of observations during the parameter-specific assessment seasons. The aspect of spatial representability in the confidence assessment is considered by a general and a specific spatial confidence aspect and both are based on a gridded approach. The accuracy of the parameter result indicates how certain the assessment is in relation to the variability of the data. For combined confidence aspects, the final confidence is taken by averaging or weighted averaging of temporal, spatial and accuracy confidence (Figure o and Table e).
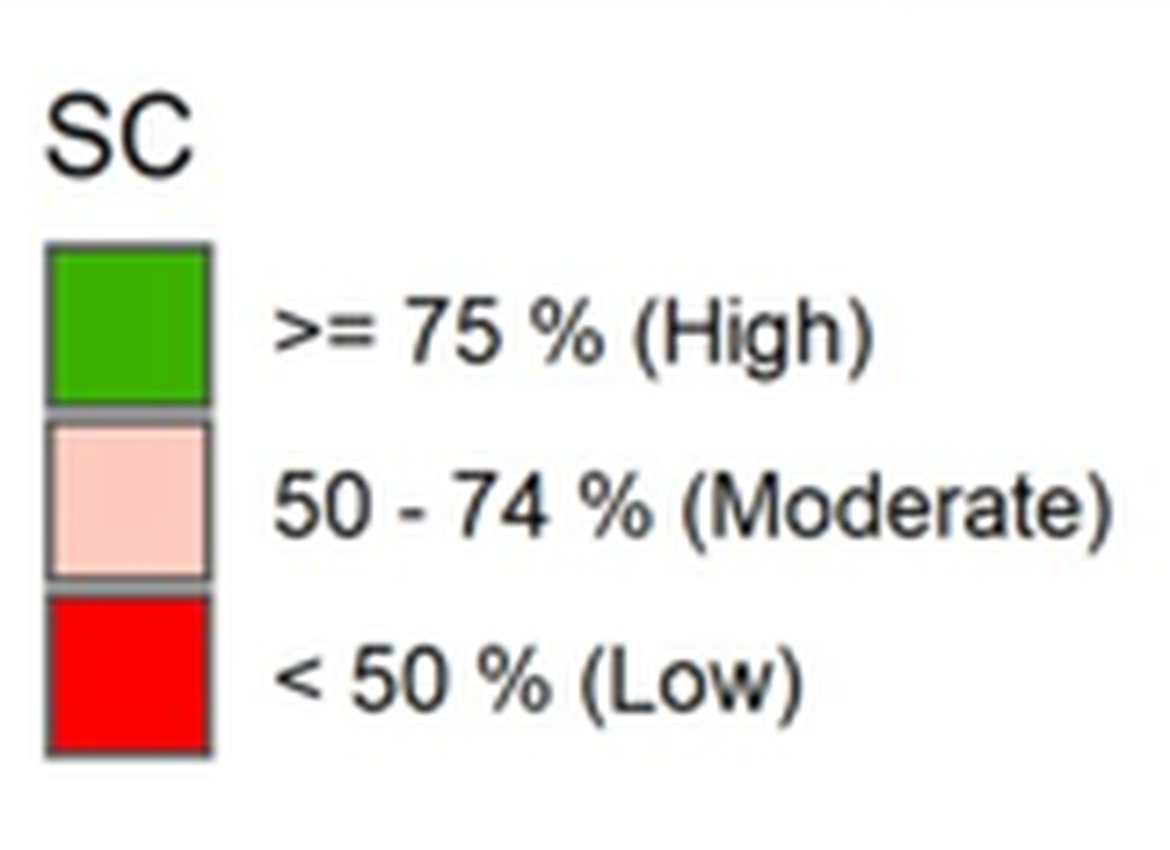
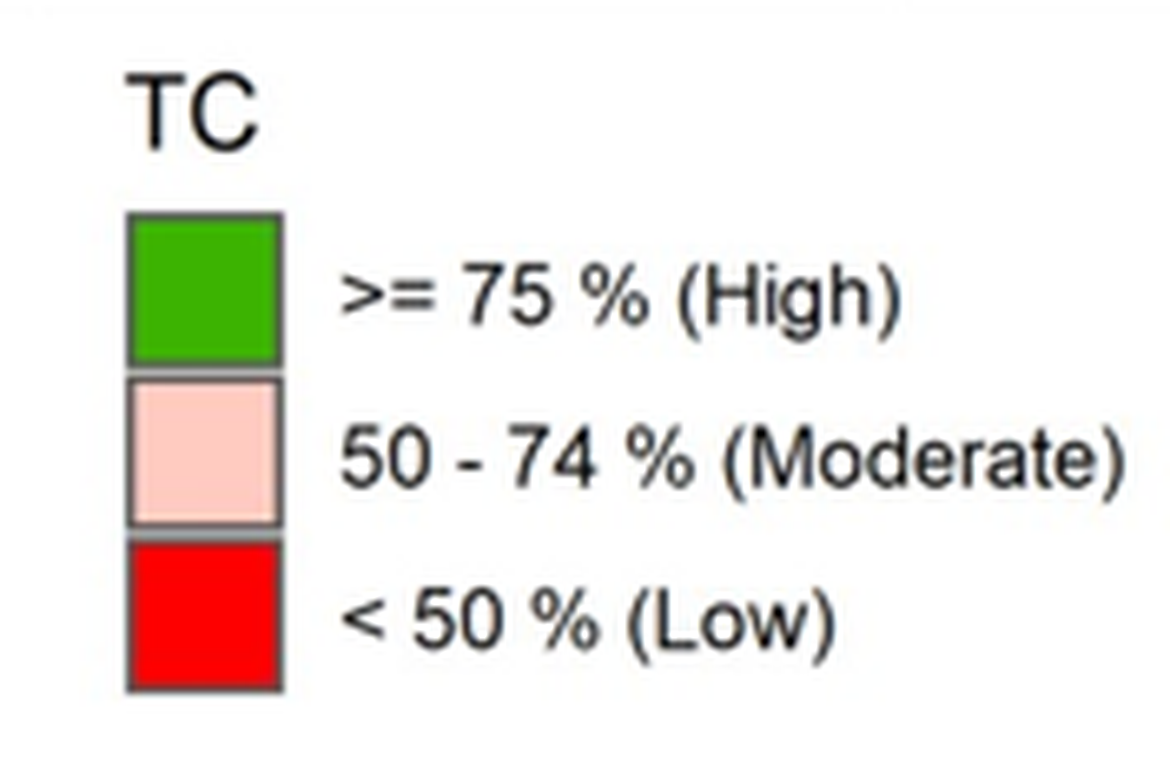
Code | ES | SD | EQR | N_OBS | TC | SC | ACC | C |
---|---|---|---|---|---|---|---|---|
ADPM | 4.4 | 0.4 | 1 | Low | Low | |||
ASS | 6.3 | 0.9 | 0.6 | 170 | Low | Low | High | Mod |
ATL | 6.6 | 0.9 | 0.7 | 26 | Low | Low | High | Low |
CCTI | 9.7 | 0.8 | 1.0 | 67 | Low | High | High | High |
CFR | 9.6 | 0.8 | 0.9 | 161 | Mod | High | High | High |
CIRL | 6.6 | 0.7 | 1 | Low | Low | |||
CNOR3 | 8.1 | 0.1 | 0.8 | 4 | Low | Low | High | Low |
CODEDK | 6.1 | 0.7 | 0.6 | 340 | High | High | High | High |
CUK1 | 7.9 | 1.1 | 0.8 | 11 | Low | Low | High | Low |
CUKC | 9.1 | 1.1 | 0.9 | 34 | Low | Low | High | Mod |
CWBC | 8.8 | 0.9 | 3 | Low | Low | |||
CWM | 8.0 | 1.6 | 0.8 | 141 | Low | Mod | High | Mod |
CWMTI | 9.3 | 1.1 | 0.9 | 134 | Mod | High | High | High |
DB | 7.5 | 0.4 | 0.8 | 68 | Low | Mod | High | Mod |
ECPM2 | 7.8 | 0.8 | 1 | Low | Low | |||
ELPM | 6.9 | 0.5 | 0.7 | 149 | Mod | Mod | High | High |
EMPM | 6.8 | 0.5 | 0.7 | 65 | Low | Low | High | Mod |
ENS | 5.7 | 0.6 | 0.6 | 678 | Mod | High | High | High |
GBC | 6.5 | 0.3 | 0.7 | 233 | Mod | High | High | High |
GBCW | 5.6 | 1.6 | 0.6 | 11 | Low | Mod | Mod | Low |
GBSW | 5.9 | 0.9 | 0.6 | 20 | Low | Low | Mod | Low |
GDPM | 5.3 | 2.4 | 0.5 | 2 | Low | Low | Low | Low |
HPM | 8.2 | 0.8 | 0.8 | 5 | Low | Low | High | Low |
IRS | 7.5 | 0.9 | 0.8 | 17 | Low | Low | High | Low |
IS1 | 7.1 | 0.2 | 0.7 | 26 | Low | Low | High | Low |
IS2 | 7.7 | 0.3 | 0.8 | 35 | Low | Low | High | Mod |
KC | 3.9 | 0.7 | 0.4 | 440 | High | High | High | High |
KD | 4.7 | 0.7 | 0.5 | 324 | High | High | High | High |
LBPM | 8.4 | 0.8 | 1 | Low | Low | |||
MPM | 6.2 | 1.1 | 0.6 | 25 | Mod | Mod | Mod | Mod |
NAAC2 | 7.0 | 0.3 | 0.7 | 23 | Low | Mod | High | Mod |
NAAC3 | 6.7 | 0.1 | 0.7 | 12 | Low | Low | High | Mod |
NNS | 6.5 | 0.5 | 0.7 | 1252 | Mod | Mod | High | High |
NT | 7.7 | 0.3 | 0.8 | 102 | High | Low | High | High |
RHPM | 7.4 | 0.2 | 0.7 | 23 | Mod | Mod | High | Mod |
SCHPM1 | 6.6 | 0.6 | 0.7 | 32 | Mod | Low | High | Mod |
SCHPM2 | 6.7 | 0.8 | 0.7 | 3 | Low | Mod | High | Mod |
SK | 6.8 | 0.4 | 0.7 | 193 | High | High | High | High |
SNS | 7.1 | 0.2 | 0.7 | 489 | High | High | High | High |
SPM | 9.7 | 0.7 | 1.0 | 34 | Low | Low | High | Mod |
SS | 7.5 | 0.3 | 0.8 | 98 | Low | Low | High | Mod |
THPM | 7.5 | 0.2 | 0.8 | 22.0 | Low | Low | High | Low |
Grid assessment
Two scales of data aggregation were adopted for the assessment, the OSPAR COMP4 areas, and smaller grids. The smaller scale of rectangles helps to identify localised areas that may be characterised by low oxygen levels (‘hotspots’). The DO assessment was applied to each grid section using data collected between July and October calculating the 5th percentile of deepest sample within 10 m of the seabed. Mean EQR values were calculated for each year, then averaged for the final EQR value. Analyses showed that mean near-bed oxygen concentrations in the 5th percentile of the data during the summer stratification season were >6mg/l in most grid cells in each region, except in the Adour Plume (1 cell), Atlantic Seasonally Stratified (4 cells), Atlantic (2 cells), Eastern North Sea (1 cell), Gulf of Biscay Coastal (7 cells), Gulf of Biscay Shelf Waters (6 cells), Gironde Plume (1 cell), Kattegat Coastal (15 cells) and Kattegat Deep (10 cells) (Figure p). Confidence assessments show clearly that assessment at the grid scale is problematic due to the many data gaps at that smaller spatial scale (Figure q).
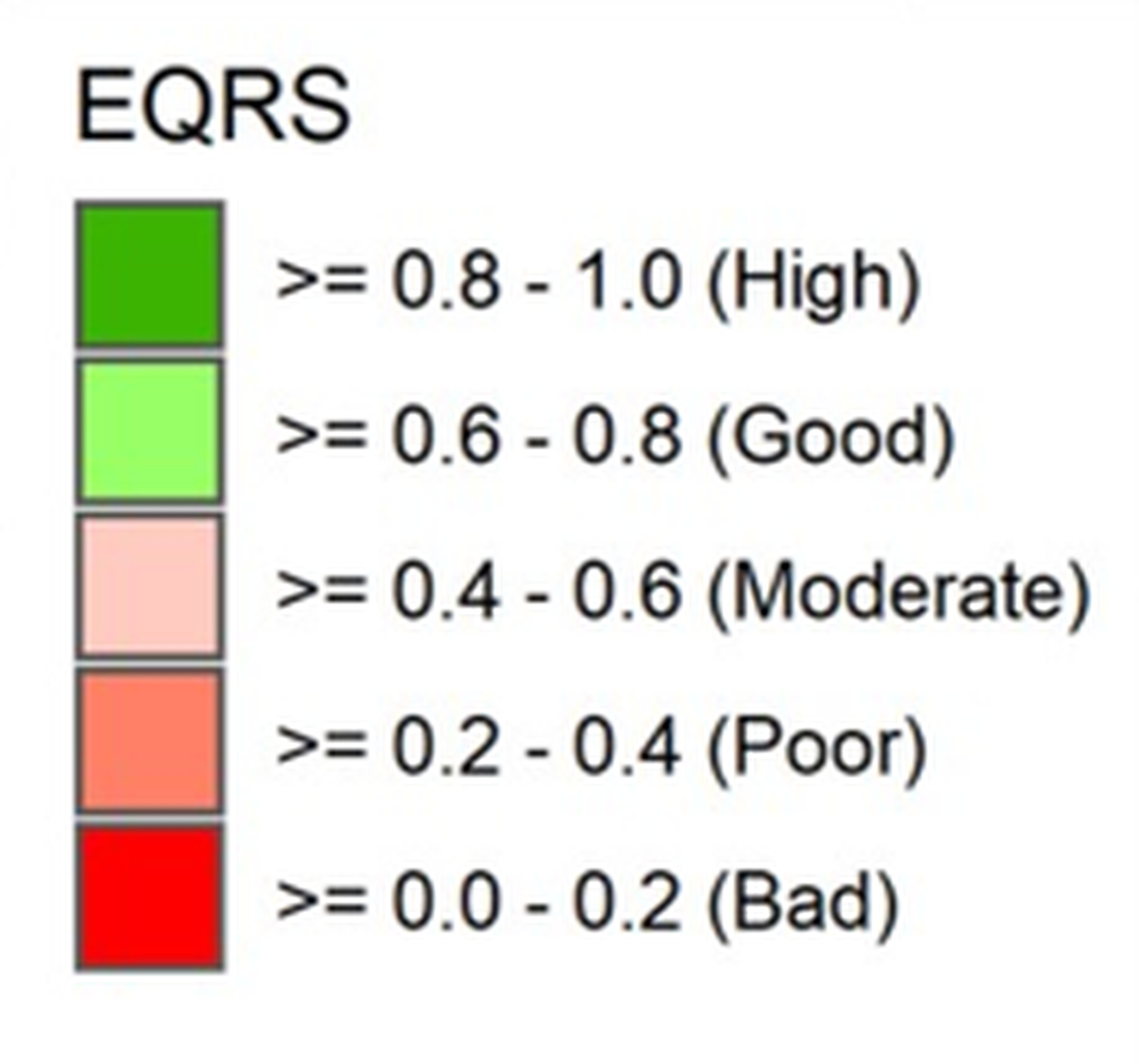
Figure p: DO assessment at smaller spatial scales. Available at: https://odims.ospar.org/en/submissions/ospar_dissolved_oxygen_eqrs_2020_08/
Figure q: Temporal confidence, accuracy class confidence and overall class confidence at the Grid scale for the DO assessment
QSR 2023
Identifying common categories of assessment areas which can align will help both the thematic assessment and the QSR. Four categories of water types (river plumes, coastal areas, shelf areas, and oceanic areas) will be used for the QSR 2023 in associating specific COMP4 assessment units with the integration categories for eutrophication, pelagic habitats and food-webs to ensure coherence across the assessments and to be consistent with MSFD requirements. As with common indicators for nutrients and Chl-1, the DO trend outcomes are categorised within each broad category (river plumes, coastal areas, shelf areas, oceanic areas) (Table f).
Area category | No Areas | Areas with data | COMP4 classification | Increasing trend | No trend | Decreasing trend |
---|---|---|---|---|---|---|
River plumes | 14 | 12 | M/P/B status | 0 | 2 | 0 |
H/G status | 0 | 8 | 2 | |||
Coastal areas | 27 | 19 | M/P/B status | 1 | 1 | 0 |
H/G status | 0 | 17 | 0 | |||
Shelf areas | 17 | 16 | M/P/B status | 0 | 3 | 0 |
H/G status | 0 | 11 | 2 | |||
Oceanic areas | 6 | 5 | M/P/B status | 0 | 0 | 0 |
H/G status | 0 | 4 | 1 |
The trend analysis was carried out on data from 1990 to 2020. Many of the assessment areas had sufficient long-term data (52 out of 64), to apply a trend assessment. Out of those 52 areas, only one in coastal areas had an increasing trend (Kattegat Coastal). Areas with decreasing trend for dissolved oxygen include two in river plumes (Meuse Plume, Scheldt Plume 1), two in Shelf areas (Norwegian Trench, Atlantic Seasonally Stratified) and one in oceanic areas (Atlantic). The Thames Plume also showed a decreasing trend but was not significant.
COMP assessment periods compared
The assessment results for the previous COMP periods, applying the COMP4 thresholds, are shown in Figure r with confidence of assessment.
In the COMP1 period (1990-2000), 4 assessment areas were classified in Moderate, Poor or Bad Status out of 36 areas. The assessment areas with eutrophication problems are mainly found in OSPAR Region II (Greater North Sea), Kattegat Coastal, Kattegat Deep, Noratlantic Area NOR-NorC2(D5) and Sudatlantic Area SUD-C1(D5). However, for the latter area the spatial and temporal confidence of the assessment is classified as Low.
In the COMP2 period (2001-2006) 5 out of 34 assessment areas were in Moderate, Poor or Bad Status, including Kattegat Coastal, Kattegat Deep, Eastern North Sea, German Bight and Coastal DEDK. In the COMP3 period (2006-2014) this was the case for 8 out of 31 assessment areas. These include Kattegat Coastal, Kattegat Deep, Eastern North Sea, German Bight and Coastal DEDK again, with the addition of Atlantic Seasonally Stratified, Channel well mixed tidal influenced and Ems Plume. In summary, the recurrent pattern over the entire period from 1990 to 2020 is, that in OSPAR Region II Greater North Sea, a number of assessment areas are classified as Moderate, Poor or Bad Status repeatedly over the entire period.
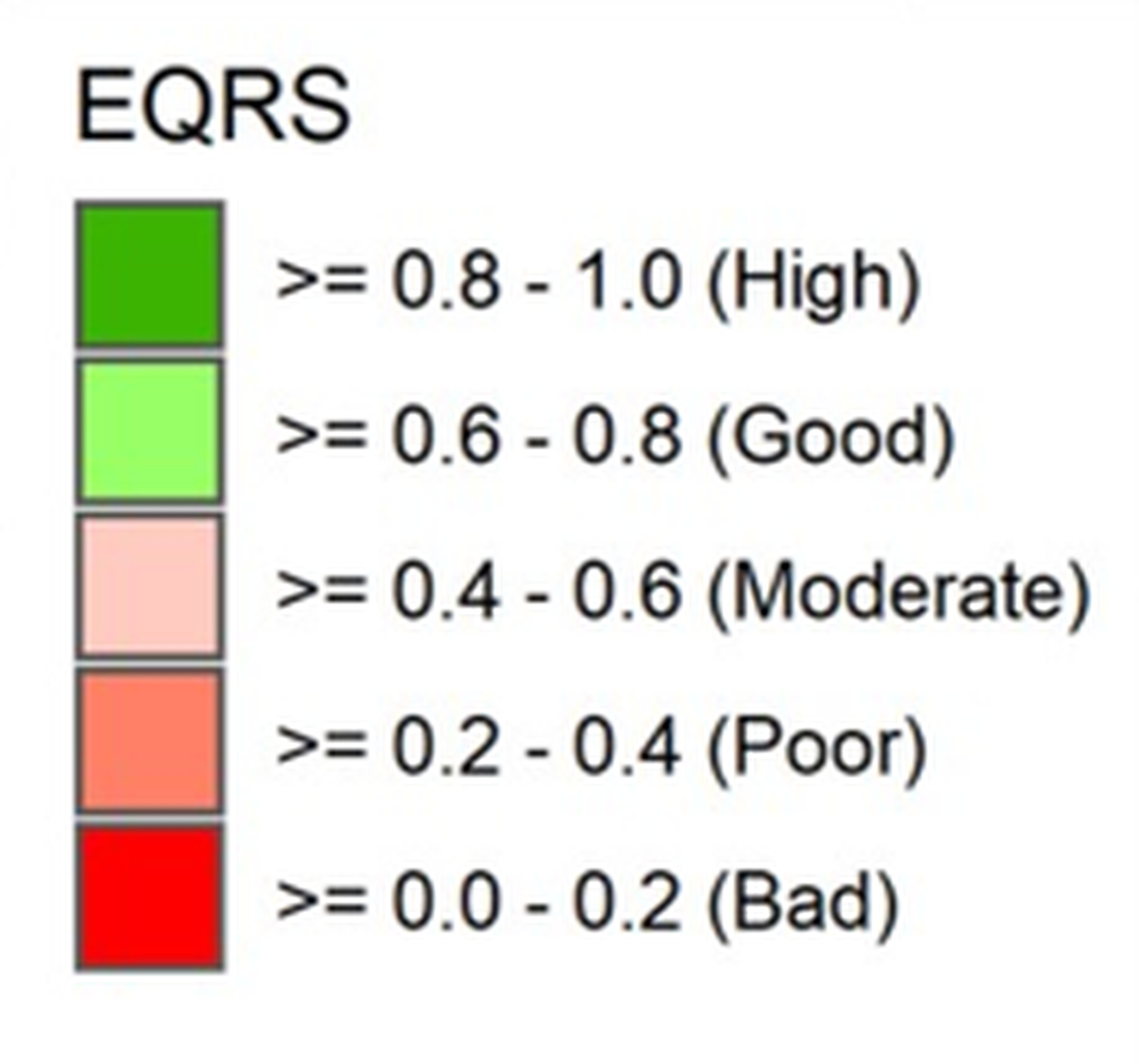
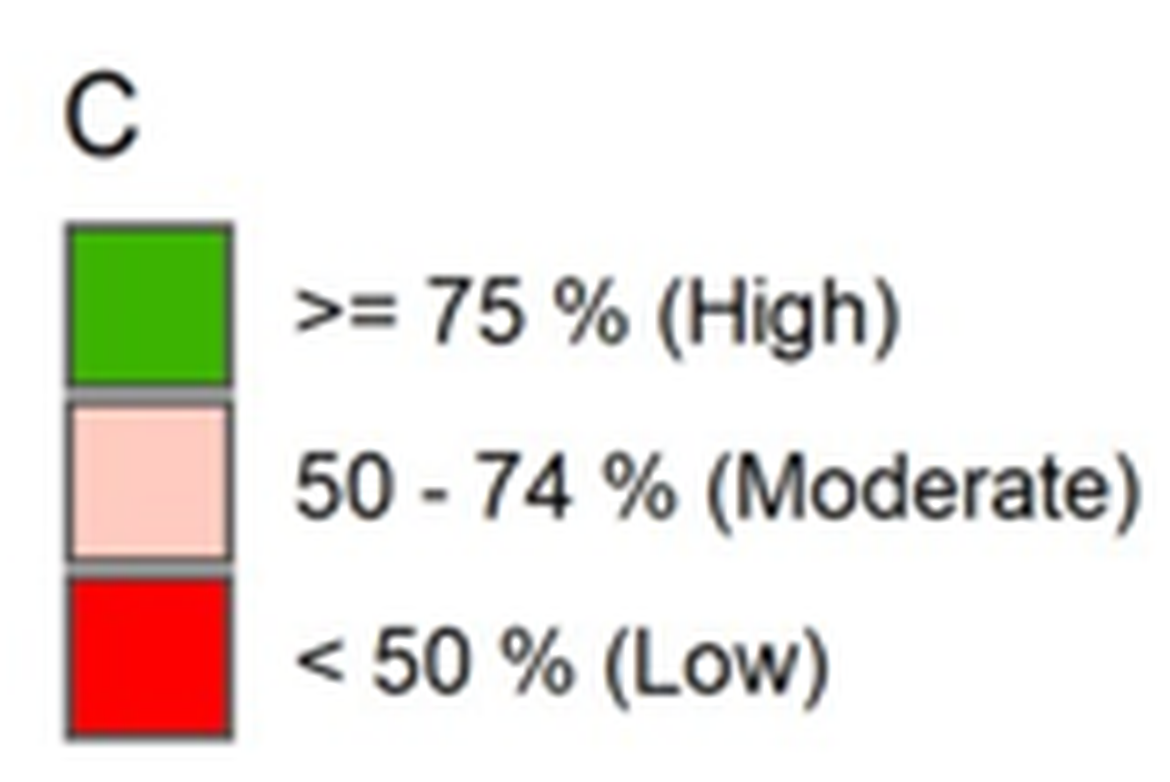
Figure r: Comparison of DO outcomes over the four assessment periods. Top panel is the assessment outcomes with measure of confidence in bottom panel. Four assessment periods are COMP1 (1990-2000), COMP2 (2001-2006), COMP3 (2006-2014) and COMP4 (2015-2020).
These changes in assessment outcomes are showing in terms of the number of assessment areas and the surface area that are identified as moderate, poor or bad outcomes for each of the COMP periods (Figure s). The number of assessment areas identified as moderate, poor or bad have increased between COMP1 and COMP4, with COMP1 (4), COMP2 (5), COMP3 (8) and COMP4 (7). The surface area covered has also increased, particularly from COMP1 (16 657km2) and COMP2 (98 318km2) to a maximum in COMP3 of 337 696km2, reducing to 110 261km2 in the current COMP4. The peak in COMP3 is influenced by the inclusion of the large area of Atlantic Seasonally Stratified (5th percentile value = 5,79mg/l). However, confidence in the assessment is low in this area for COMP3.
Figure s: Number of areas and surface area of the assessment areas that are moderate, poor or bad over the four COMP periods
Comparison of the four COMP assessment periods shows that plume and shelf waters have declined with coastal waters varying between 10 to 20%. Oceanic waters always achieved good or high status over the four comp periods (Figure t).
Figure t: Percentage of the surface area of river plumes, coastal waters, shelf areas and oceanic waters in Bad, Poor or Moderate Status (red) or Good or High status (green) in the COMP1, COMP2, COMP3 and COMP4 assessment periods. Grey bars indicate assessment areas with missing data
Conclusion
Across most of the assessment areas of the OSPAR COMP regions, there is no widespread oxygen deficiency. However, localised areas of oxygen deficiency are apparent, particularly in the Eastern North Sea, Kattegat and Gulf of Biscay. Oxygen concentrations and percentage saturation are improving in the Kattegat and deteriorating in a localised area of the south-eastern North Sea. The Skagerrak, Kattegat and Sound have lower mean concentrations, but are showing improvements over time.
Across most of the assessment areas of the OSPAR COMP regions, there is no widespread oxygen deficiency. However, localised areas of oxygen deficiency are apparent, particularly in the Eastern North Sea, Kattegat and Gulf of Biscay. Oxygen concentrations and percentage saturation are improving in the Kattegat and deteriorating in a localised area of the south-eastern North Sea. The Skagerrak, Kattegat and Sound have lower mean concentrations, but are showing improvements over time.
There are statistically significant trends in reducing oxygen concentration in 5 regions (Atlantic, Atlantic Seasonally Stratified, Meuse, Scheldt Plume, Norwegian Trench) and one increasing in oxygen concentration (Kattegat Coastal). Of these regions it is only the Atlantic Seasonally Stratified region where the reduced solubility due to increased sea temperature is the dominant effect.
Climate change and dissolved oxygen
In European shelf seas, most areas (not Baltic) become vertically well mixed in winter every year, and as such oxygen concentrations are reset to the surface values. As oxygen consumption is significantly driven from the bed, the regions which suffer from low oxygen, are those which have a relatively small volume of water beneath the thermocline. Climate change can impact upon the dissolved oxygen concentration in many ways. Most evidently via the direct effect on solubility but it can also increase stratification which inhibits the supply of oxygenated waters to lower levels. Increased levels of CO2 can promote phytoplankton growth above or on the thermocline, which when it decays and is consumed beneath the thermocline can decrease oxygen concentrations. Increased bottom water temperature can also increase bacterial consumption enhancing draw down.
Climate and the oceans are very closely linked, with climate change having serious effects on marine ecosystems. It is estimated that the amount of the gas dissolved in the oceans declined by 2% between 1960 and 2010, though there are very strong regional variations, with many factors at play.
Knowledge Gaps
Understanding of the relative importance of biological and physical processes (e.g., mixing and currents) in controlling near-bed oxygen dynamics, and the impacts of climate change on physical processes, oxygen deficiency and oxygen consumption is complex, with multiple interactions between the physical and biological processes.
Further work on the depth of the seabed investigating what is the most appropriate depth level exploring where the minimum DO would be located as the very low DO may not be picked up with current methods.
Need for better data to capture the high spatial and temporal variability, e.g., using automated sampling devices and supported by modelling.
Development in assessment methods (e.g., including models, looking at number of days below threshold).
Understanding the relative importance of biological and physical processes (e.g., mixing and currents) in controlling near-bed oxygen dynamics, and the impacts of climate change on physical processes, oxygen deficiency and oxygen consumption is complex, with multiple interactions between the physical and biological processes. Recent studies have focused on distinguishing between the effects of nutrient enrichment and changes in seawater temperature as a result of climate change. Furthermore, robust assessments of oxygen deficiency require improved availability of suitable data. Since oxygen deficiency is localised and often short-lived, modelling can help in identifying hotspots see below example.
The Atlantic Sea Seasonally Stratified region, which comprises much of the Celtic Sea, is one area which has poor coverage by observations. Biogeochemical models (Ciavatta, 2016; van Leeuwen et al., 2015), which replicate the observations well, show that regions of low oxygen concentrations are likely to occur over quite extensive regions, however not in the regions where sampling presently occurs. Models not only have a role in directing future sampling programmes and strategies to make best use of resources, but in the future may if suitably validated be used as evidence in regions which are poorly sampled at present.
Validated biogeochemical models have the capability to assess the impact of targeted reduction scenarios on oxygen concentrations in problem areas, noting that there is often a spatial disconnect between nutrient sources and the areas impacted (Lehmann et al., 2014). There are also potentially significant time lags in the system, so that modelling can help inform how long a management measure will have to persist before an outcome becomes apparent.
Further work on the depth of the seabed investigating what is the most appropriate depth level exploring where the minimum DO would be located as the very low DO may not be picked up with current methods (Ærtebjerg et al. 2002; Erlandsson et al., 2006). It also reduces the available data for the assessment. Seabed changes can occur close to the seabed with 10 m potentially being too far from where the changes are being measured. The 10 m was chosen for pragmatic reasons to pick up the bottom of profiles where they do not go too close to the seabed, so we need to be sure that this method is sufficient to pick up DO sag issues. Profile and glider data will allow us to explore this in detail. The very low DO may not be picked up with the current methods.
Key knowledge gaps include understanding of the impacts of climate change on hydrodynamic processes and oxygen deficiency. Improved understanding of the impacts of climate change requires further research (Greenwood et al., 2010; Queste et al., 2013, 2016; Topcu and Brockmann, 2015; Große et al., 2016; Hansen et al., 2013a, 2013b) and assessments based on oxygen saturation levels. Assessments based on saturation levels require oxygen measurements to be paired with temperature and salinity measurements so that percentage saturation can be calculated. Robust assessments of oxygen deficiency require improved availability of suitable data in many of the assessment areas. While the initial International Council for the Exploration of the Sea (ICES) dataset included good spatial and temporal coverage, the dataset was much reduced when it was filtered to obtain the specific data required for the assessments, such as from near the seafloor and for the duration of the stratification period (1 July–31 October).
The ICES database includes measurements from all depths. The application of filters (e.g., depth, season, and salinity) reduces the dataset considerably, highlighting the need for targeted monitoring for future assessments. The observation that many of the available data points were in nearshore waters highlights the importance of improved spatial coverage in order to improve confidence in assessments of marine areas. Furthermore, recent publications (e.g., Greenwood et al., 2010; Queste et al., 2013, 2016; Topcu and Brockmann, 2015; Große et al., 2016), which indicate the availability of additional data not yet included in the ICES database, highlight the need for existing monitoring and / or research data, which are held in local or regional databases, to be uploaded to ICES. The methodology, developed over many years and refined for this assessment, requires some further development to address questions about the feasibility and practicalities of near-bed monitoring and assessment.
Ærtebjerg G, Carstensen J, Axe P, et al (2002) The 2002 Oxygen Depletion Event in the Kattegat, Belt Sea and Western Baltic. Baltic Sea Environment Proceedings No. 90. Helsinki Comm 66 pp
Artioli, Yuri, Jana Friedrich, Alison J. Gilbert, Abigail McQuatters-Gollop, Laurence D. Mee, Jan E. Vermaat, Fred Wulff, Christoph Humborg, Luca Palmeri, and Falk Pollehne. "Nutrient budgets for European seas: a measure of the effectiveness of nutrient reduction policies." Marine Pollution Bulletin 56, no. 9 (2008): 1609-1617.
Barry J and Maxwell D (2015) Tools for environmental and ecological survey design and analysis. Available at: https://r-forge.r-project.org/projects/emon/
Best M, Wither AW, Coates S (2007) Dissolved oxygen as a physico-chemical supporting element in the Water Framework Directive. Mar Poll Bull 55:53–64. Available at: https://doi.org/10.1016/j.marpolbul.2006.08.037
Brockmann, U. H., and K. Eberlein. "River input of nutrients into the German Bight." In The role of freshwater outflow in coastal marine ecosystems, pp. 231-240. Springer Berlin Heidelberg, 1986.
Brockmann, U., Billen, G. and Gieskes, W.W., 1988. North Sea nutrients and eutrophication. Pollution of the North Sea: an assessment, pp.348-389.
Ciavatta, S., Kay, S., Saux-Picart, S., Butenschon, M. and Allen, J.I. (2016) Decadal reanalysis of biogeochemical indicators and fluxes in the North West European shelf-sea ecosystem. Journal of Geophysical Research: Oceans. 121. Available at: https://doi.org/10.1002/2015JC011496
Chan, Francis, J. A. Barth, J. Lubchenco, A. Kirincich, H. Weeks, William T. Peterson, and B. A. Menge. "Emergence of anoxia in the California current large marine ecosystem." Science 319, no. 5865 (2008): 920-920.
Conley, D. J. (2002). Terrestrial ecosystems and the global biogeochemical silica cycle. Global Biogeochemical Cycles,16(4), 68-1.
European Council Directive 91/676/EEC of 12 December 1991 concerning the protection of waters against pollution caused by nitrates from agricultural sources
ECJ 2009. European Court of Justice Judgment of the Court (Third Chamber) of 10 December 2009. European Commission v United Kingdom of Great Britain and Northern Ireland. Failure of a Member State to fulfil obligations – Environment – Directive 91/271/EEC – Urban waste water treatment - Article 3(1) and (2), Article 5(1) to (3) and (5) and Annexes I and II – Initial failure to identify sensitive areas – Concept of ‘eutrophication’ – Criteria – Burden of proof – Relevant date when considering the evidence – Implementation of collection obligations – Implementation of more stringent treatment of discharges into sensitive areas. Case C-390/07 European Court Reports 2009 I-00214
EPA and Marine Institute (2016) Ireland National Eutrophication Report under the Common Procedure, 2016. ICG EUT 17/4/Info.3. Compiled by the Environmental Protection Agency and the Marine Institute. 53 pp
Erlandsson CP, Stigebrandt A, Arneborg L (2006) The sensitivity of minimum oxygen concentrations in a fjord to changes in biotic and abiotic external forcing. Limnol Oceanogr 51:631–638. Available at: https://doi.org/10.4319/lo.2006.51.1_part_2.0631
Greenwood N, Parker ER, Fernand L, et al (2010). Detection of low bottom water oxygen concentrations in the North Sea; implications for monitoring and assessment of ecosystem health. Biogeosciences 7:1357-1373
Große F, Greenwood N, Kreus M, et al (2016) Looking beyond stratification: a model-based analysis of the biological drivers of oxygen depletion in the North Sea. Biogeosciences Discuss 12:12543–12610. doi: 10.5194/bgd-12-12543-2015. Available at: http://www.biogeosciences.net/13/2511/2016/
Gröger, Matthias, Ernst Maier-Reimer, Uwe Mikolajewicz, Andreas Moll, and Dmitry Sein. "NW European shelf under climate warming: implications for open ocean–shelf exchange, primary production, and carbon absorption." Biogeosciences 10, no. 6 (2013): 3767-3792.
Hansen JLS, Bendtsen J (2013a) Increasing hypoxia in the North Sea – Baltic Sea transition zone in a future warmer climate. 2013.
Hansen JLS, Bendtsen J (2013b) Parameterisation of oxygen dynamics in the bottom water of the Baltic Sea-North Sea transition zone. Mar Ecol Prog Ser 481:25–29. doi: 10.3354/meps10220
Kendall, MG (1975) Rank correlation methods,4th ed.; Charles Griffith: London.
Lehmann A, Hinrichsen HH, Getzlaff K, Myrberg K (2014) Quantifying the heterogeneity of hypoxic and anoxic areas in the Baltic Sea by a simplified coupled hydrodynamic-oxygen consumption model approach. J Mar Syst 134:20–28. Available at: https://doi.org/10.1016/j.jmarsys.2014.02.012
Levin L, Ekau W, Gooday AJ, et al (2009) Effects of natural and human-induced hypoxia on coastal benthos. Biogeosciences 2063–2098. https://doi.org/10.5194/bgd-6-3563-2009
Mann HB (1945) Non-parametric tests against trend. Econometrica, 13, 245-259.
Meire LK, Soetaert KE, Meysman FJ. Impact of global change on coastal oxygen dynamics and risk of hypoxia. Biogeosciences. 2013 Apr 19;10(4):2633-53.
Montgomery, HoAoCo, N. S. Thom, and A. Cockburn. "Determination of dissolved oxygen by the Winkler method and the solubility of oxygen in pure water and sea water." Journal of Applied Chemistry 14, no. 7 (1964): 280-296.
Peeters, J. C. H., F. J. Los, R. Jansen, H. A. Haas, L. Peperzak, and I. De Vries. "The oxygen dynamics of the Oyster Ground, North Sea. Impact of eutrophication and environmental conditions." Ophelia 42, no. 1 (1995): 257-288.
OSPAR Agreement 2010-03. The North-East Atlantic Environment Strategy. Strategy of the OSPAR Commission for the Protection of the Marine Environment of the North-East Atlantic 2010–2020
OSPAR Agreement 2013-8. Common Procedure for the Identification of the Eutrophication Status of the OSPAR Maritime Area. Supersedes Agreements 1997-11, 2002-20 and 2005-3
OSPAR (2022). Revision of the Common Procedure for the Identification of the Eutrophication Status of the OSPAR Maritime Area. OSPAR Agreement 2022-07.
Queste BY, Fernand L, Jickells TD, Heywood KJ (2013). Spatial extent and historical context of North Sea oxygen depletion in August 2010. Biogeochemistry 113:53–68. https://doi.org/10.1007/s10533-012-9729-9
Queste BY, Fernand L, Jickells TD, et al (2016) Drivers of summer oxygen depletion in the central North Sea. Biogeosciences Discuss 12:8691–8722. https://doi.org/10.5194/bgd-12-8691-2015
SMHI (2007) Swedish National Report on Eutrophication Status in the Kattegat and the Skagerrak. OSPAR Assessment 2007. 103 pp.
SMHI (2016) Swedish National Report on Eutrophication Status in the Skagerrak, Kattegat and the Sound. OSPAR Assessment 2016. ICG EUT 17/4/Info.6. 70 pp.
Topcu HD, Brockmann UH (2015) Seasonal oxygen depletion in the North Sea, a review. Mar Pollut Bull 99:5–27. https://doi.org/10.1016/j.marpolbul.2015.06.021
van Leeuwen S, Tett P, Mills D, van der Molen J (2015) Stratified and non-stratified areas in the North Sea: Long-term variability and biological and policy implications. J Geophys Res Ocean 120:4670–4686. https://doi.org/10.1002/2014JC010485
Contributors
Lead authors: Michelle Devlin, Liam Fernand and Kate Collingridge
Supported by: Technical Group for the revision and application of the Common Procedure, Intersessional Correspondence Group on Eutrophication, Intersessional Correspondence Group on Eutrophication Modelling and Hazardous Substances and Eutrophication Committee.
Citation
Devlin, M., Fernand, L. and Collingridge, K. 2022. Concentrations of Dissolved Oxygen Near the Seafloor in the Greater North Sea, Celtic Seas and Bay of Biscay and Iberian Coast. In: OSPAR, 2023: The 2023 Quality Status Report for the North-East Atlantic. OSPAR Commission, London. Available at: https://oap.ospar.org/en/ospar-assessments/quality-status-reports/qsr-2023/indicator-assessments/seafloor-dissolved-oxygen
Assessment type | Indicator Assessment |
---|---|
Summary Results | https://odims.ospar.org/en/submissions/ospar_dissolved_oxygen_msfd_2022_06/ |
SDG Indicator | 14.1 By 2025, prevent and significantly reduce marine pollution of all kinds, in particular from land-based activities, including marine debris and nutrient pollution |
Thematic Activity | Eutrophication |
Relevant OSPAR Documentation | OSPAR Agreement 2010-03 The North-East Atlantic Environment Strategy. Strategy of the OSPAR Commission for the Protection of the Marine Environment of the North-East Atlantic 2010–2020 |
Date of publication | 2022-06-30 |
Conditions applying to access and use | https://oap.ospar.org/en/data-policy/ |
Data Snapshot | https://odims.ospar.org/en/submissions/ospar_dissolved_oxygen_snapshot_2022_06/ |
Data Results | https://odims.ospar.org/en/submissions/ospar_dissolved_oxygen_results_2022_06/ |
Data Source | https://www.ices.dk/data/data-portals/Pages/DOME.aspx |