Executive Summary
The functioning of a marine ecosystem is highly dependent on the structure, diversity and integrity of its components and their interactions. Food webs represent networks of feeding interactions between predators and their prey at different levels in the ecosystem.
Environmental and anthropogenic pressures alter the balance between organisms. Any human activity that has an impact on biota in our marine ecosystems is also likely to have an impact on food webs, and this impact can be local or far away from the site of the pressure. Trends in primary production have been mainly driven by reduced nutrient availability and increasing sea surface temperatures. The latter is the main pressure associated with trends in plankton functional groups. Trends in fish communities are mainly linked to fishing pressures. Marine food webs and the ecosystem’s carrying capacity support not only the diversity of life in our seas and oceans, but also many of the ecosystem services that humans very much depend on. Knowledge of the structure and functioning of food webs is therefore not only key for understanding marine ecosystem functioning and how it may be conserved or restored but also for facilitating sustainable use of our seas in the future.
In this thematic assessment a set of food web indicators demonstrates that the abundances, distribution and productivity of key groups representing different trophic levels are in flux in many of the assessed areas. The production of phytoplankton as well as the second layer of food webs are assessed by analysing changes in zooplankton and ratios between plankton life forms. Fish communities are also assessed, in terms of size structure, species composition and biomass of feeding guilds linking lower trophic levels to predators. The effects of fishing pressures on the structure of demersal fish were analysed by assessing the status of different trophic levels including mesopredators and top predators. Finally, the existing knowledge of all compartments, trophic levels and their interactions is integrated into an assessment of the state of the whole food web.
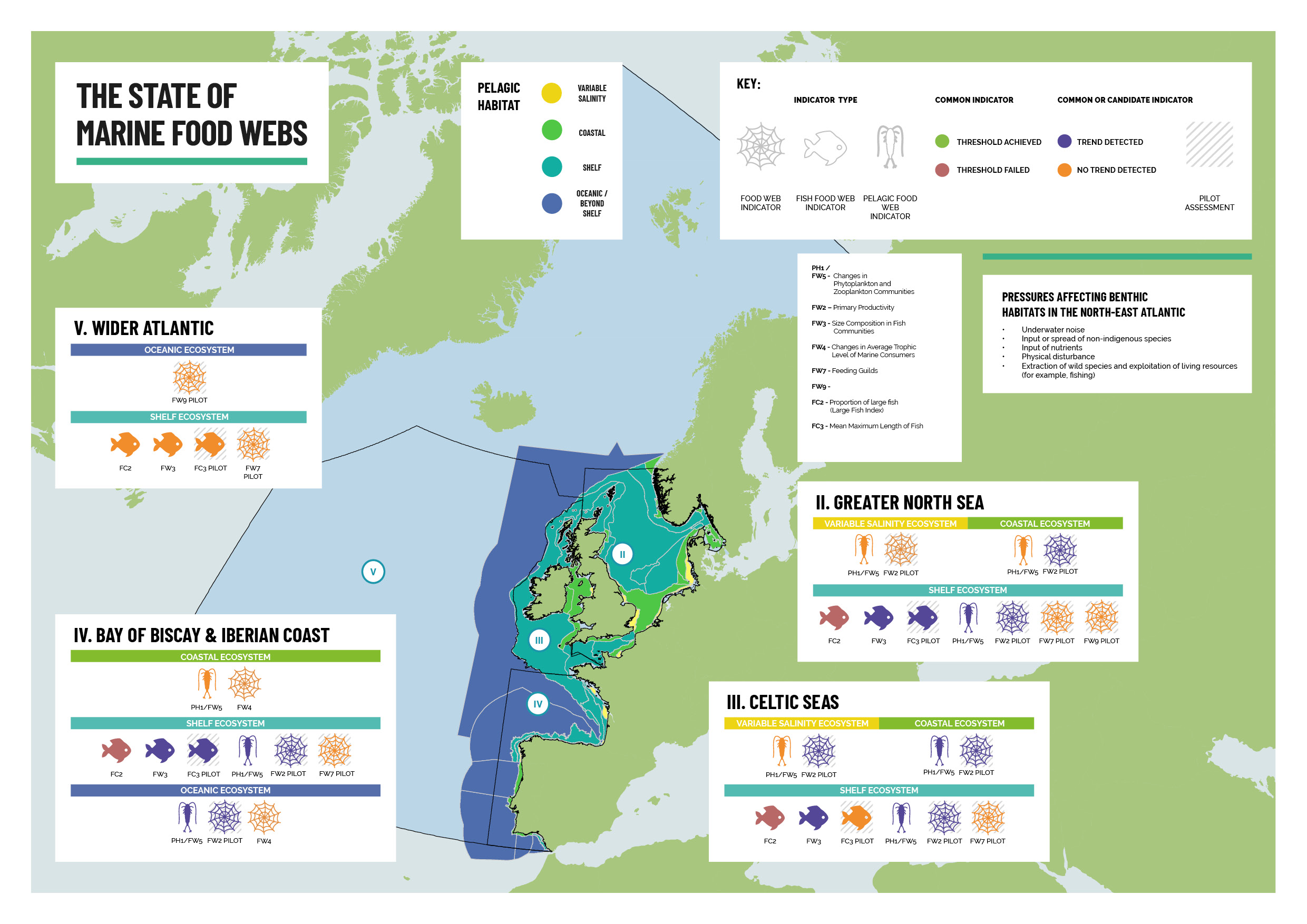
Q1. Identify the problems? Are they the same in all OSPAR Regions?
In the study of marine food webs, the distribution, productivity and abundance of all marine biota and the relationships between them are of relevance, as they in a sense integrate the status of all the ecosystem components. Given the comprehensive nature of food web status, the human activities affecting food webs span the width of those affecting the status of marine birds, marine mammals, fish, benthic and plankton communities, and non-indigenous species.
Society’s need for food results in fishing activities in the OSPAR Maritime Area, involving the extraction of commercially important species, as well as by-catch of non-commercial species. This can cause imbalances in predator-prey relationships, with consequent negative effects on the food web. Fishing can also cause physical disturbance of the seabed, impacting the benthic communities on which some fish species depend as a food source.
Society’s need for energy and to mitigate climate change, requires new infrastructure to be built in the marine environment. Construction can impact benthic and pelagic food web components through alteration of habitats and changes to hydrological and hydrodynamic conditions, with negative effects for nutrients.
Society’s need for trade and movement of goods and the associated increase in shipping can result in the translocation of non-indigenous species and introduces underwater noise. Non-indigenous species have the potential to compete with native species for space and food, affecting trophic levels and negatively affecting primary productivity.
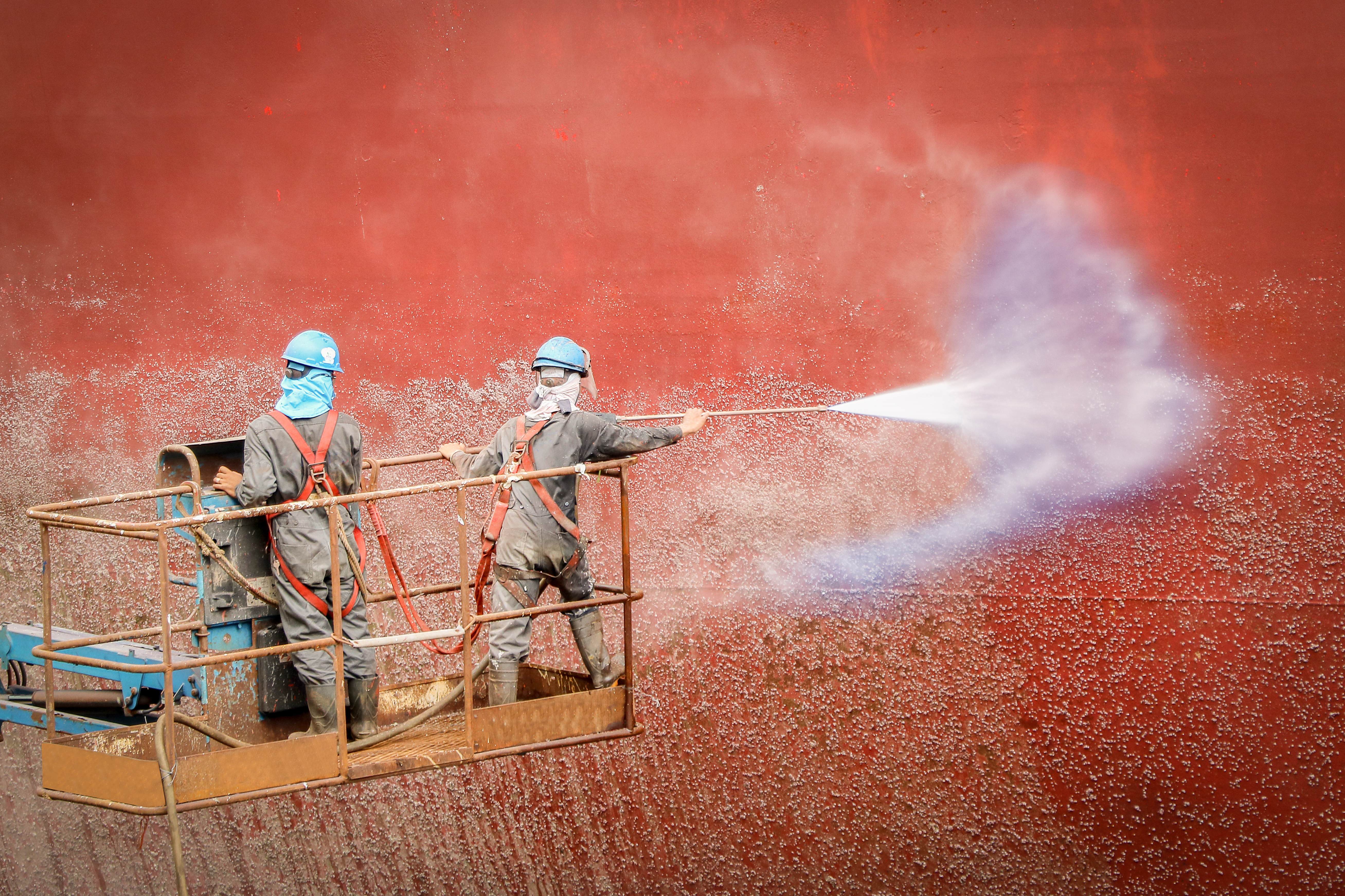
Non-indigenous species introduced by shipping have the potential to compete with native species for space and food, affecting trophic levels and negatively affecting primary productivity. © Shutterstock
Underwater noise can also impact fish and top-predator communities and alter the behaviour of marine mammals, which in turn affecting lower trophic levels.
Climate change is also affecting the food web. As the waters warm, they are changing the distribution and range of many species in the food web throughout the OSPAR Maritime Area. This has already affected the primary production rates and species composition of phytoplankton and zooplankton, which in turn will trigger changes at other trophic levels. In addition, climate change alters species phenology and life-cycle events and leads to a desynchronization of biotic interactions, in particular predator-prey relationships.
These stressors may have a greater impact in some regions than in others, but given the level of globalization in human activities, all OSPAR Regions are affected to a greater or lesser extent.
Q2. What has been done?
Food webs are the umbrella that connects all biodiversity components, from primary producers to apex predators, and their various interactions and energy transfers across trophic levels must be taken into account. This makes food webs particularly hard to investigate and assess. Understanding food web structure and changes is critical in order to formulate policy that can lead to appropriate management responses. Responses that address climate change, fishing, pollution and eutrophication have been identified as important for supporting good environmental status of food webs in the North-East Atlantic.
OSPAR has not adopted any specific measures with the explicit objective of supporting the state, function or resilience of food webs. There are, however, several examples of measures that could have particular relevance to ecosystem function and resilience. These include the OSPAR MPA network and Other Effective Conservation Measures (OECMs).
Q3. Did it work?
The ambition to apply an ecosystem approach to the management of human activities is central to the work of OSPAR, but implementation remains challenging. Even though, arguably, every measure to improve the state of the marine environment is ultimately (also) aimed at conserving or restoring food webs to good status, connecting response measures to (positive or negative) effects on food webs is complex, also because the impact is seldom direct in nature. It is critically important to continue to improve food web indicators in order to inform the development and implementation of responses that take a more integrated approach.
Q4. How does this field affect the overall quality status?
The food web is key to the health of the marine ecosystem. Significant impacts on one element of the food web can impact the whole ecosystem through knock-on and cascading effects. A well-balanced food web is a key factor underpinning a healthy marine ecosystem. A suite of indicators has therefore been used to address the differing trophic levels in the food web under a holistic approach that aims to build an overall picture of food web status. This assessment covers all OSPAR Regions, and the overall results indicate that food webs are not in good environmental status.
In the Greater North Sea, the indicators for demersal fish communities have not achieved the threshold indicating good status, which suggests that this component of the food web is under pressure. Plankton community indicators show a decreasing trend of primary production and a mixed picture for various plankton lifeforms, with some showing increasing trends and others decreasing. The fish feeding guild indicator shows an increasing trend in planktivore biomass in shelf ecosystems between 1997 to 2020. There is a mixed picture for other guilds that feed largely on benthos and fish: their biomass generally shows increasing trends in the southern part of the Greater North Sea and decreasing trends in its northern part. The multitrophic level indicator demonstrates that primary productivity has been the main driver of changes in trophic functioning in coastal ecosystems. Different trends were observed in Ecological Network Analysis-related indices, resulting in unknown status for the trophic network.
In the Celtic Seas, the indicators for demersal fish communities have not achieved the thresholds indicating good status, suggesting a long term status decline in the Region. Plankton community indicators show a decreasing trend for primary production and for virtually all plankton lifeforms. The fish feeding guild indicator shows an increasing trend in the biomass of three of the four guilds investigated in the northern part of the Region. Contrasting trends in the biomass of fish that feed largely on the benthos were found, with generally increasing trends in the southern part of the Celtic Seas and decreasing trends in the northern part of the Region.
In the Bay of Biscay and Iberian Coast, the indicators for demersal fish communities demonstrate no clear patterns. Top predators and mesopredators show signs of recovery although they display contrasting trends at sub-division level, which implies unknown status. Plankton community indicators show a significant downward trend in primary production, and a mixture of upward and downward trends for different plankton lifeforms. These decreasing trends can be linked to rising sea surface temperatures. The fish feeding guild indicator shows that the biomass of fish guilds is generally highest in the northern part of the Region.
In the Wider Atlantic, the indicators for demersal fish communities demonstrate no long term changes. The fish feeding guild indicator shows that benthivore biomass was the same in the two survey areas. Planktivore and piscivore biomass are generally highest in the southern area. The pilot assessment with the multitrophic level indicator conducted in the area of the Azores shows a decreasing trend for biomass at all trophic levels in this food web model. Changes to the indices in modelled ecosystem network analyses indicate that, for the area studied in this pilot assessment, the resilience of the ecosystem is decreasing over time.
A different but equivalent approach was taken in Arctic Waters, where the Common Indicators were not available. This assessment used evidence taken from other sources (Arctic Council / AMAP / ICES) and indicates that climate change is currently the strongest driver of change in the marine food web in this Region.
The food web assessment results indicate potential risks of both bottom-up and top-down effects impacting ecosystem function.
Q5. What do we do next?
The NEAES 2030 operational objective SX.O2 commits OSPAR to initiating discussions on the development of a practical approach for regional-scale ecosystem-based management, including through the Collective Arrangement and in cooperation with fishery management bodies and other competent organisations. The objective is to strengthen ecosystem resilience to climate change and to safeguard the marine environment, its biodiversity and ecosystem services. Progress in the implementation of this objective will be an important contribution to developing appropriate responses for food webs.
The development of a practical approach to ecosystem-based management (EBM) will provide the opportunity and the mechanism to share evidence and common objectives for more sustainable use of the marine environment. Working with interested partners and drawing on international best practice, OSPAR will design and implement a pilot project on EBM in one of the OSPAR Regions. In addition, more knowledge of the potential future impacts of windfarms on trophic structure and food web functioning is needed.
There are opportunities for OSPAR to strengthen its response to support food webs, including by taking a broader ecosystem approach to the implementation of actions to strengthen the status of OSPAR listed species and habitats, further developing the OSPAR MPA network, including OECMs, and implementing the strategic objectives which address eutrophication. Strengthening cooperation with those bodies that have competence for managing human activities outside of OSPAR’s mandate will be critical to success.
Ecosystem restoration provides another potential opportunity to support healthy food webs in line with NEAES Strategic Objective 6, to “Restore degraded benthic habitats in the North-East Atlantic when practicable to safeguard their ecosystem function and resilience to climate change and ocean acidification”.
With regard to the OSPAR MPA network, understanding the management effectiveness of MPAs within the network, and the network itself, remains an important gap to address, particularly from an ecosystem function and food-web perspective. By 2022, OSPAR aimed to identify barriers to the effective management of MPAs, and by 2024 it will take appropriate steps to enable all OSPAR MPAs to achieve their conservation objectives (NEAES S5.O2). The contribution of OECMs and improvement in the management of MPAs within and outside national jurisdiction will be an important response for food webs.
For OSPAR MPAs in Areas Beyond National Jurisdiction (ABNJs), continued effort will be needed to further the Collective Arrangement (OSPAR Agreement 2014-09) and to cooperate through other mechanisms, such as Memoranda of Understanding, with relevant competent management authorities to help them consider appropriate management actions for delivering the conservation objectives for OSPAR MPAs in ABNJs.
Atwood, T. B. and Hammill, E. (2018). The Importance of Marine Predators in the Provisioning of Ecosystem Services by Coastal Plant Communities. Frontiers in Plant Science, 9(1289). doi:10.3389/fpls.2018.01289
Baird, D., Asmus, H. and Asmus R. (2012). Effect of invasive species on the structure and function of the Sylt-Rømø Bight ecosystem, northern Wadden Sea, over three time periods. Marine Ecology Progress Series 462,143-162.
Baird, D., Asmus, H., Asmus, R., Horn, S. and De la Vega, C. (2019). Ecosystem response to increasing ambient water temperatures due to climate warming in the Sylt-Romo Bight, northern Wadden Sea, Germany. Estuarine, Coastal and Shelf Science 228, 106322
Beaugrand, G., Edwards, M., Raybaud, V., Goberville, E. and Kirby, R.R. (2015). Future vulnerability of marine biodiversity compared with contemporary and past changes. Nature Climate Change, 5(7), pp.695-701.
Blanchard, J.L., 2015. A rewired food web. Nature, 527(7577), pp.173-174.
Bruge, A., Alvarez, P., Font´an, A., Cotano, U. and Chust, G. (2016). Thermal niche tracking and future distribution of Atlantic mackerel spawning in response to ocean warming. Front. Mar. Sci. 3 https://doi.org/10.3389/fmars.2016.00086.
Burrows, M.T., Schoeman, D.S., Buckley, L.B., Moore, P., Poloczanska, E.S., Brander, K. M., Brown, C., Bruno, J.F., Duarte, C.M., Halpern, B.S., Holding, J., Kappel, C.V., Kiessling, W., O’Connor, M.I., Pandolfi, J.M., Parmesan, C., Schwing, F.B., Sydeman, W.J. and Richardson, A.J. (2011). The pace of shifting climate in marine and terrestrial ecosystems. Science 334 (6056), 652–655.
Burthe, S., Daunt, F., Burler, A., Elston, D.A., Frederikson, M. et al. (2012). Phenological trends and trophic mismatch across multiple levels of a North Sea pelagic food web. Marine Ecology Progress Series 454: 119-133
Capuzzo, E., Lynam, C. P., Barry, J., Stephens, D., Forster, R. M., Greenwood, N., McQuatters-Gollop, A., Silva, T., van Leeuwan, S. M. and Engelhard, G. H. (2018). A decline in primary production in the North Sea over 25 years, associated with reductions in zooplankton abundance and fish stock recruitment. Global change biology, 24(1), e352-e364.
Chapman, E.J., Byron, C.J., Rasher-Lasley, R., Lipsky, C., Stevens, J.R. and Peters, R. (2020). Effects of climate change on coastal ecosystems food webs: implications for aquaculture. Marine Environemental Research 162, https://doi.org/10.1016/j.marenvres.2020.105103
Cheung, W.W., Lam, V.W., Sarmiento, J.L., Kearney, K., Watson, R., Pauly, D. (2009). Projecting global marine biodiversity impacts under climate change scenarios. Fish Fish. 10 (3), 235-251
Chust, G., González, M., Fontán, A., Revilla, M., Alvarez, P., Santos, M., Cotano, U., Chifflet, M., Borja, A., Sagarminaga, Y., Caballero, A., de Santiago, I., Epelde, I., Liria, P., Ibaibarriaga, L., Garnier, F., Franco, J., Villarino, E., Irigoien, X., Fernandes-Salvador, J. A., Uriarte, A., Esteban, X., Orue-Echervarria, D., Figueira, T. and Uriarte, A. (2022). Climate regime shifts and biodiversity redistribution in the Bay of Biscay. Science of The Total Environment, 803, 149622.
Daunt, F., Wanless, S., Greenstreet, S.P.R., Jensen, H., Hamer, K.C., Harris, M.P. (2008) The impact of the sandeel fishery closure on seabird food consumption, distribution, and productivity in the northwestern North Sea. Canadian Journal of Fisheries and Aquatic Sciences 65: 362-381
Duarte, C. M., Hendriks, I. E., Moore, T. S., Olsen, Y. S., Steckbauer, A., Ramajo, L., Carstensen, J., Trotter, J. A. & McCulloch, M. (2013). Is ocean acidification an open-ocean syndrome? Understanding anthropogenic impacts on seawater pH. Estuaries and Coasts, 36(2), 221-236.
Dulvy, N.K., Rogers, S.I., Jennings, S., Stelzenmüller, V., Dye, S.R. and Skjoldal, H.R. (2008). Climate change and deepening of the North Sea fish assemblage: a biotic indicator of warming seas. J. Appl. Ecol. 45 (4), 1029–1039
European Commission (2018). REPORT FROM THE COMMISSION TO THE EUROPEAN PARLIAMENT AND THE COUNCIL assessing Member States' programmes of measures under the Marine Strategy Framework Directive (COM/2018/562 final) accessed https://eur-lex.europa.eu/legal-content/EN/TXT/PDF/?uri=CELEX:52018DC0562&from=EN
Eriksson, B., Sieben, K., Eklöf, J., Ljunggren, L., Olsson, J., Casini, M. and Bergström, U. (2011). Effects of Altered Offshore Food Webs on Coastal Ecosystems Emphasize the Need for Cross-Ecosystem Management. Ambio, 40, 786-797. doi:10.1007/s13280-011-0158-0
Fossheim, M., Primicerio, R., Johannesen, E., Ingvaldsen, R.B., Aschan, M.M. and Dolgov, A. V. (2015). Recent warming leads to a rapid borealization of fish communities in the Arctic. Nat. Clim. Change 5 (7), 673–677
Gehlen, M., Séférian, R., Jones, D.O., Roy, T., Roth, R., Barry, J., Bopp, L., Doney, S.C., Dunne, J.P., Heinze, C. and Joos, F. (2014). Projected pH reductions by 2100 might put deep North Atlantic biodiversity at risk. Biogeosciences, 11(23), pp.6955-6967.
Goldenberg, S.U., Nagelkerken, I., Ferreira, C.M., Ullah, H. and Connell, S.D. (2017). Boosted food web productivity through ocean acidification collapses under warming. Global change biology 23: 4177-4184
Greenstreet, S. P. R., Rogers, S. I., Rice, J. C., Piet, G. J., Guirey, E. J., Fraser, H. M. and Fryer, R. J., (2011). Development of the EcoQO for the North Sea fish community. ICES Journal of Marine Science 68: 1–11.
Greenstreet, S.P.R., Armstrong, E., Mosegaard, H., Jensen, H., Gibb, I., Fraser, H., Scott, B., Holland, G. and Sharples, J. (2006). Variation in the abundance of sand eels Ammodytes marinus off southeast Scotland: an evaluation of area-closure fisheries management and stock abundance assessment methods. ICES J. Mar. Sci. 63: 1530-1550
Heath, M.R., Speirs, D.C. and Steel, J.H. (2014). Understanding patterns and processes in models of trophic cascades. Ecologica letters, 17(1), 101-114
Hernvann, P. Y., Gascuel, D., Grüss, A., Druon, J. N., Kopp, D., Perez, I., Piroddi, C. and Robert, M. (2020). The Celtic Sea through time and space: Ecosystem modeling to unravel fishing and climate change impacts on food-web structure and dynamics. Frontiers in Marine Science, 1018.
Hofstede, T.R., Hiddink, J.G. and Rijnsdorp, A.D. (2010). Regional warming changes fish species richness in the eastern North Atlantic Ocean. Mar. Ecol. Prog. Ser. 414, 1–9.
Holt, J., Allen, J. I., Anderson, T. R., Brewin, R., Butenschön, M., Harle, J., Huse, G., Lehodey, P., Lindemann, C., Memery, L., Salohoglu, B., Senina, I. and Yool, A. (2014). Challenges in integrative approaches to modelling the marine ecosystems of the North Atlantic: Physics to fish and coasts to ocean. Progress in Oceanography, 129, 285-313
Horta e Costa, B., Assis, J., Franco, G., Erzini, K., Henriques, M., Gonçalves, E.J. and Caselle, J.E., 2014. Tropicalization of fish assemblages in temperate biogeographic transition zones. Mar. Ecol. Prog. Ser. 2014 (504), 241.
Jepsen, J.U., Arneberg, P., Ims, R.A., Siwertsson, A. and Yoccoz, N.G. (2020). Panel-based Assessment of Evosystem Condition (PAEC) – Technical protocol version2. NINA Report 1980. Norwegian Institute for Nature Research
Johannesen E., Yoccoz, N., Tveraa, T., Schackell, N.L., Ellingsen, K., Dolgov, A.V. and Frank, K.T., (2020). Resource-driven colonization by cod in a high Arctic food web. Ecology and Evolution 10: 14272–14281. DOI: https://doi.org/10.1002/ece3.7025
Jung, A.S., van der Veer, H.W., Philippart, C.J.M., Waser, A.M., Ens, B.J., de Jonge, V.N. and Schückel, U. (2020). Impacts of macrozoobenthic invasions on a temperate coastal food web. Marine Ecology Progress Series 653: 19-39.
Kleisner, K.M., Fogarty, M.J., McGee, S., Hare, J.A., Moret, S., Perretti, C.T. and Saba, V.S. (2017). Marine species distribution shifts on the U.S. Northeast Continental Shelf under continued ocean warming. Prog. Oceanogr. 153, 24–36.
Kortsch, S., Primicerio, R., Fossheim, M., Dolgov, A.V. and Aschan, M. (2015). Climate change alters the structure of arctic marine food webs due to poleward shifts of boreal generalists. Proceedings of the Royal Society B: Biological Sciences, 282(1814), p.20151546.
Lawrence, M.J., Stemberger, H.L.J., Zolderdo, A.J., Struthers, D.P. and Cooke, S.J. (2015). The effects of modern war and military activities on biodiversity and the environment. Environ. Rev 23: 443-460
Lenoir, S., Beaugrand, G. and Lecuyer, E. (2011). Modelled spatial distribution of marine fish and projected modifications in the North Atlantic Ocean. Glob. Change Biol. 17, 115–129.
Le Marchand, M. Ben Rais Lasram, F., Araignous, E., Saint-Béat, B., Lassalle, G., Michelet, N., Serre, S., Safi, G., Lejart, M., Niquil, N. and Le Loc´h, F. (2022). Potential combined impacts of climate change and non-indigenous species arrivals on Bay of Biscay trophic network structure and functioning. Journal of Marine Systems 228, https://doi.org/10.1016/j.jmarsys.2022.103704
Levin, L.A. and Le Bris, N. (2015). The deep ocean under climate change. Science, 350(6262), pp.766-768.
Levin, L.A., Wei, C.L., Dunn, D.C., Amon, D.J., Ashford, O.S., Cheung, W.W., Colaço, A., Dominguez‐Carrió, C., Escobar, E.G., Harden‐Davies, H.R. and Drazen, J.C. (2020). Climate change considerations are fundamental to management of deep‐sea resource extraction. Global change biology, 26(9), pp.4664-4678.
Lynam, C.P., Llope, M., Möllmann, C., Helaouët, P., Bayliss-Brown, G.A. and Stenseth, N.C. (2017). Interaction between top-down and bottom-up control in marine food webs. Proceedings of the National Academy of Sciences 114 (8): 1952-1957
Machado, I., Costa L.J.and Cabral, H. (2021). Response of food-webs indicators to human pressures, in the scopre of the Marine Strategy Framework Directive. Front. Mar.Sci., https://www.frontiersin.org/articles/10.3389/fmars.2021.699566/full
Meyer, J., Nehmer, P., Moll, A.and Kröncke, I. (2018). Shifting south-eastern north Sea macrofauna community structure since 1986: A response to de-eutrophication and regionally decreasing food supply? Estuarine, Coastal and Shelf Science 213: 115-127
Morato, Telmo, José‐Manuel González‐Irusta, Carlos Dominguez‐Carrió, Chih‐Lin Wei, Andrew Davies, Andrew K. Sweetman and Gerald H. Taranto. (2020). "Climate‐induced changes in the suitable habitat of cold‐water corals and commercially important deep‐sea fishes in the North Atlantic." Global Change Biology 26, no. 4: 2181-2202.
Machado I., Costa J.L., and Cabral, H. (2021). Response of Food-Webs Indicators to Human Pressures, in the Scope of the Marine Strategy Framework Directive. Front. Mar. Sci. 8:699566. doi: 10.3389/fmars.2021.699566
Morato, T., Lemey, E., Menezes, G., Pham, C.K., Brito, J., Soszynski, A., Pitcher, T.J. and Heymans, J.J. (2016). Food-web and ecosystem structure of the open-ocean and deep-sea environments of the Azores, NE Atlantic. Frontiers in Marine Science, 3, p.245.
Nogues, Q., Raoux, A., Araignous, E., Chaalali, A., Hattab T., Leroy, B., Ben Rais Lasram, F., David, V., Le Loc’h, F., Dauvin, J.C. and Niquil, N. (2021). Cumulative effects of marine renewable energy and climate change on ecosystem properties: Sensitivity of ecological network analysis. Ecological Indicators, 121, 107128.
Nogues, Q., Araignous, E., Bourdaud, P., Halouani, G. and Raoux, A. (2022). Spatialized ecological network analysis for ecosystem-based management: effects of climate change, marine renewable energy, and fishing on ecosystem functioning in the Bay of Seine. ICES Journal of Marine Science 79: 1098-1112
Odum E. P. 1969 Science, New Series, Vol. 164, No. 3877. pp. 262-270
Olbert, A. I., Dabrowski, T., Nash, S. and Hartnett, M. (2012). Regional modelling of the 21st century climate changes in the Irish Sea. Continental Shelf Research, 41, 48-60.
Perez, F.F., Fontela, M., García-Ibáñez, M.I., Mercier, H., Velo, A., Lherminier, P., Zunino, P., De La Paz, M., Alonso-Pérez, F., Guallart, E.F. and Padin, X.A. (2018). Meridional overturning circulation conveys fast acidification to the deep Atlantic Ocean. Nature, 554(7693), pp.515-518.
Piet G. J., Tamis J. E., Volwater J., de Vries P., van der Wal J. T. and Jongbloed, R. H. (2021). A Roadmap Towards Quantitative Cumulative Impact Assessments: Every Step of the Way. Sci. Total Environ. 784, 146847. doi: 10.1016/j.scitotenv.2021.146847
Piroddi, C., Akoglu, E., Andonegi, E., Bentley, J. W., Celić, I., Coll, M. and Tsikliras, A. C. (2021). Effects of nutrient management scenarios on marine food webs: a Pan-European Assessment in support of the Marine Strategy Framework Directive. Frontiers in Marine Science, 8, 179.Pitois SG, Graves CA, Close H, Lynam C, Scott J, Tilbury J, van der Kooij J, Culverhouse P. (2021). A first approach to build and test the Copepod Mean Size and Total Abundance (CMSTA) ecological indicator using in-situ size measurements from the Plankton Imager (PI), Ecological Indicators 123, 107307. https://doi.org/10.1016/j.ecolind.2020.107307
Puerta, P., Johnson, C, Carreiro-Silva, M., Henry, LA, Kenchington, E., Morato, T., Kazanidis, G., Jose Luis Rueda, J.L., Urra, J., Ross, S., Wei, C.-L., González-Irusta J.M., Arnaud-Haond, S. and Orejas, C. (2020). Influence of water masses on the biodiversity and biogeography of deep-sea benthic ecosystems in the North Atlantic. Frontiers in Marine Science 7, 239.
Punzón, A., Serrano, A., Sánchez, F., Velasco, F., Preciado, I., González-Irusta, J. and López-López, L. (2016). Response of a temperate demersal fish community to global warming. J. Mar. Syst. 161, 1–10.
Punzón A., López-López L., González-Irusta J.M., Preciado I., Hidalgo M., Serrano A., Tel E., Somavilla R., Polo J., Blanco M., Ruiz-Pico S., Fernández-Zapico O., Velasco F.and Massutí, E., (2020). Tracking the effect of temperature in marine demersal fish communities. Ecological indicators. https://doi.org/10.1016/j.ecolind.2020.107142
Queirós, A.M., Fernandes, J., Genevier, L. and Lynam, C.P. (2018). Climate change alters fish community size-structure, requiring adaptive policy targets. Fish and Fisheries 2018: 1-9, https://doi.org/10.1111/faf.12278
Righton, D.A., Andersen, K.H., Neat, F., Thorsteinsson, V., Steingrund, P., Svedang, H., Michalsen, K., Hinrichsen, H.H., Bendall, V., Neuenfeldt, S., Wright, P., Jonsson, P., Huse, G., van der Kooij, J., Mosegaard, H., Hüssy, K. and Metcalfe, J. (2010). Thermal niche of Atlantic cod Gadus morhua: limits, tolerance and optima. Mar. Ecol. Prog. Ser. 420, 1–13.
Rossoll D, Bermu´dez R, Hauss H, Schulz KG and Riebesell, U. (2012) Ocean Acidification-Induced Food Quality Deterioration Constrains Trophic Transfer. PLoS ONE 7(4): doi:10.1371/journal.pone.0034737
Safi, G., Giebels, D., Arroyo, L., Heymans, J., Preciado, I., Raoux, A., Schückel, U., Tecchio, S., de Jonge V.N. and Niquil, N. (2019). Vitamine ENA: A framework for the development of ecosystem-based indicators for decision-makers. Ocean, Coastal and Management 174:116-130
Simpson, S.D., Jennings, S., Johnson, M.P., Blanchard, J.L., Schön, P.-J., Sims, D.W. and Genner, M.J. (2011). Continental shelf-wide response of a fish assemblage to rapid warming of the sea. Curr. Biol. 21, 1565–1570
Soler, G.A., Edgar, G.J., Thomson, R.J., Kininmonth, S., Campbell, S.J. and Dawson, T.P. (2015). Reef Fishes at All Trophic Levels Respond Positively to Effective Marine Protected Areas. PLoS ONE10(10): e0140270. doi:10.1371/journal.pone.0140270. Available from: https://www.researchgate.net/publication/282943302_Reef_Fishes_at_All_Trophic_Levels_Respond_Positively_to_Effective_Marine_Protected_Areas [accessed May 30 2022].
Spence, M.A., Griffiths, C.A., Waggitt, J.J., Bannister, H.J., Thorpe, R.B., Rossberg, A.G. and Lynam, C.P. (2021). Sustainable fishing can lead to improvements in marine ecosystem status: an ensemble-model forecast of the North Sea ecosystem. Marine Ecology Progress Series 680:207-221. https://doi.org/10.3354/meps13870
Spence, M.A., Lynam, C.P., Thorpe, R.B., Heneghan, R.F. and Dolder, P.J. (2022). Synthesizing Empirical and Modelling Studies to Predict Past and Future Primary Production in the North Sea. Frontiers in Marine Science 9:828623. https://doi.org/10.3389/fmars.2022.828623
Sweetman, A.K., Thurber, A.R., Smith, C.R., Levin, L.A., Mora, C., Wei, C.L., Gooday, A.J., Jones, D.O., Rex, M., Yasuhara, M. and Ingels, J. (2017). Major impacts of climate change on deep-sea benthic ecosystems. Elementa: Science of the Anthropocene, 5.
Tavares, D. C., Moura, J. F., Acevedo-Trejos, E. and Merico, A. (2019). Traits Shared by Marine Megafauna and Their Relationships with Ecosystem Functions and Services. Frontiers in Marine Science, 6(262). doi:10.3389/fmars.2019.00262
Thompson, M.S.A., Pontalier, H., Spence, M., Pinnegar, J.K., Greenstreet, S., Moriarty, M., Hélaouët, P. and Lynam, P.C. (2020). A feeding guild indicator to assess environmental change impacts on marine ecosystem structure and functioning. Journal of Applied Ecology 57 (9): 1769-1781, https://doi.org/10.1111/1365-2664.13662
Thorpe, R.B., Arroyo, N.L., Safi, G., Niquil, N., Preciado, I., Heath, M., Pace, M.C. and Lynam, C.P. (2022). The Response of North Sea Ecosystem Functional Groups to Warming and Changes in Fishing. Frontiers in Marine Science 9:841909. https://doi.org/10.3389/fmars.2022.841909
Tilstone, G. H., Land, P. E., Pardo, S., Kerimoglu, O. and Van der Zande, D. (2022). Threshold indicators of primary production in the north-east Atlantic for assessing environmental disturbances using 21 years of satellite ocean colour. Science of The Total Environment, 158757.
Ulanowicz, R. E. (2004). Quantitative methods for ecological network analysis. Computational biology and chemistry 28: 321–339
Ullah, H., Nagelkerken, I., Goldenberg, S.U. and Fordham, D.A. (2018). Climate change could drive marine food web collapse through altered trophic flows and cyanobacterial proliferation. PLOS Biology 16(1): e2003446. https://doi.org/10.1371/journal.pbio.2003446
Woodstock, M.S. and Zhang, Y. (2022). Towards ecosystem modeling in the deep sea: A review of past efforts and primer for the future. Deep Sea Research Part I: Oceanographic Research Papers, p.103851.
Xavier, J., Carreiro-Silva, M., Colaço, A., Le Bris, N. and Levin, L. (2019). Chapter 6. Vulnerabilities: Invertebrate taxa (VME indicators), In Deep-ocean climate change impacts on habitat, fish and fisheries, by Lisa Levin, Maria Baker, and Anthony Thompson (eds). FAO Fisheries and Aquaculture Technical Paper No. 638. Rome, FAO. 186 pp. Licence: CC BY-NC-SA 3.0 IGO.
Food webs Assessments
Contributors
Lead authors: Ulrike Schückel, Izaskun Preciado
Supporting authors: Christopher Lynam, Murray Thompson, Ger-Jan Piet, Joey Volwater, Federico Cornacchia, Rob van der Veeren, Quentin Nogues, Nathalie Niquil, Joana Brito, Telmo Morato, Emily Corcoran, Maria A. Torres, Gro I. van der Meeren, Eva Varkevisser, Matthew Holland, Arnaud Louchart, Abigail McQuatters-Gollop and Luis Felipe Artigas
Supported by: Intersessional Correspondence Group on the Coordination of Biodiversity Assessment and Monitoring (OCG-COBAM), Biodiversity Committee (BDC), Intersessional Correspondence Group on the Quality Status Report (ICG-QSR), Intersessional Correspondence Group on Ecosystem Assessment Outlook (ICG-EcoC), Intersessional Correspondence Group on Economic and Social Analysis (ICG-ESA), and OSPAR Commission Secretariat
Citation
OSPAR, 2023. Food webs Thematic Assessment. In: OSPAR, 2023: Quality Status Report 2023. OSPAR Commission, London. Available at: https://oap.ospar.org/en/ospar-assessments/quality-status-reports/qsr-2023/thematic-assessments/food-webs/