Tendances des efflorescences de l’espèce phytoplanctonnique nocive phaeocystis dans les eaux belges, néerlandaises et allemandes
D5 - Eutrophisation
D5.2 - Effets directs de l’enrichissement en nutriments
Message clé:
On n’a relevé aucune tendance temporelle des efflorescences annuelles de l’espèce phytoplanctonnique nocive phaeocystis le long des côtes belges, néerlandaises et allemandes; elles sont cependant très abondantes.
Contexte
L'objectif stratégique d’OSPAR, en ce qui concerne l’eutrophisation, consiste à lutter contre l'eutrophisation dans la zone maritime OSPAR, dans le but ultime de parvenir à, et de maintenir, un milieu marin sain exempt d’eutrophisation anthropique. Les tendances des efflorescences de l’espèce phytoplanctonnique nocive phaeocystis sont l’un des cinq indicateurs de l’eutrophisation. Cette série d’indicateurs peut identifier l’eutrophisation lorsque ces indicateurs sont évalués et étudiés ensemble dans le cadre de la Procédure Commune d’OSPAR selon une méthode à plusieurs étapes.
Un enrichissement excessif en nutriments des eaux marines peut entraîner des efflorescences algales (phytoplancton) et donc, éventuellement, des perturbations indésirables de l’équilibre des organismes dans les écosystèmes marins et de la qualité de l’eau en général. Les perturbations indésirables sont notamment des modifications de la composition et de l’ampleur de la flore et de la faune et l’appauvrissement en oxygène causés par la décomposition de matière organique accumulée produite par le phytoplancton ou les communautés algales durant leurs saisons de croissance. Les phaeocystis sont un phytoplancton marin répandu. Lorsqu’elles se dégradent, à la fin d’une floraison, de la mousse peut se former. Elles servent d’indicateur de l’eutrophisation car des teneurs en hausse de plus de 106 ou 107 de cellules de phaeocystis par litre d’eau de mer et des efflorescences de phaeocystisdurant plus longtemps par an indiquent un enrichissement en nutriments.
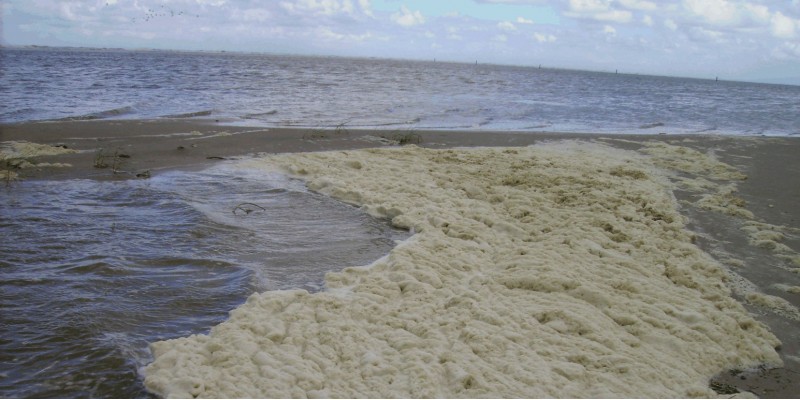
Présence de phaeocystis sur la plage de Spiekeroog, Allemagne ©Wera Leujak, Umweltbundesamt
Eutrophication is the result of excessive enrichment of water with nutrients. This may cause accelerated growth of algae and / or higher forms of plant life (EC, 1991). This may result in an undesirable disturbance to the balance of organisms present and thus to overall water quality. Undesirable disturbances can include shifts in the composition and extent of flora and fauna and the depletion of oxygen due to decomposition of accumulated biomass. Such disturbances then have other effects, such as changes in habitats and biodiversity, blooms of nuisance algae or macroalgae, a decrease in water clarity, and behavioural changes or even death of fish and other species. Identifying causal links between these disturbances and nutrient enrichment can be complicated by other pressures. Cumulative effects, including climate change, may have similar effects on biological communities and dissolved oxygen, further complicating efforts to demonstrate causal links.
The OSPAR Commission’s strategic objective, with regard to eutrophication, is to combat eutrophication in the OSPAR Maritime Area, with the ultimate aim that by 2020 the OSPAR Maritime Area is a healthy marine environment where anthropogenic eutrophication does not occur (OSPAR, 2010). The OSPAR Eutrophication Strategy requires assessment of eutrophication to be based on the ecological consequences of nutrient enrichment and not just on nutrient enrichment alone, i.e. finding reliable evidence for accelerated growth of algae and / or macrophytes caused by anthropogenic nutrient enrichment, leading to undesirable disturbance. Eutrophication is diagnosed using OSPAR’s harmonised criteria of nutrient inputs, concentrations and ratios, chlorophyll-a concentrations, phytoplankton indicator species, macrophytes, dissolved oxygen levels, incidence of fish kills and changes in zoobenthos (OSPAR, 2013-8). As there is no single indicator of disturbance caused by marine eutrophication, OSPAR applies a multi-step method using the harmonised criteria. Eutrophication is considered to have occurred if there is evidence for all of the stages shown in Figure a and of causal links between them (ECJ, 2009).
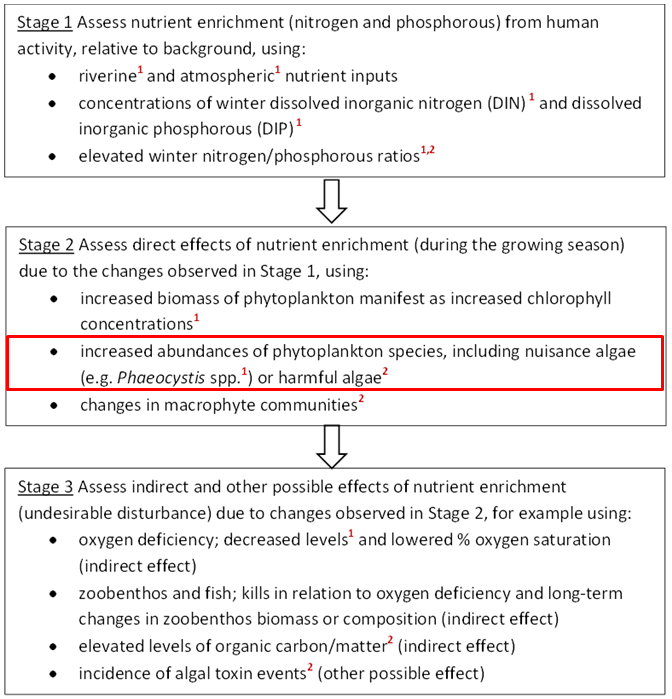
Figure a: Three stages in the identification of eutrophication. The criteria marked ¹ are common indicators for the OSPAR Intermediate Assessment 2017.
The criteria marked ² are not relevant in all OSPAR countries’ waters. The common indicator assessed here (concentrations of the nuisance algae Phaeocystis) is outlined in red
For OSPAR’s Intermediate Assessment 2017, five harmonised criteria have been assessed at a regional scale: nutrient inputs, nutrient concentrations and ratios, chlorophyll-a concentrations, concentrations of the nuisance algae Phaeocystis, and dissolved oxygen levels. These are highlighted in Figure a. The individual assessment results of any one of these five common indicators do not diagnose eutrophication by themselves. However, the assessments provide useful information about trends and are important for informing management measures.
Phaeocystis was assessed in the Quality Status Report (QSR) 2010 as part of the Common Procedure. There is no comparable regional indicator assessment of Phaeocystis.
Currently six species are assigned to the genus Phaeocystis, of which three are associated with bloom formation. In the southern North SeaPhaeocystis species consist of P. globosa (flagellates and non-flagellates) and P. pouchetti, which could partly not be distinguished. For this reason only cell concentrations of Phaeocystis species are reported. Phaeocystis has a polymorphic life cycle, alternating between free-living cells and gelatinous colonies comprising thousands of cells embedded in a polysaccharidic matrix. The colonies range from several millimetres (Rousseau et al., 1994; Peperzak et al., 2000b) up to 3 cm (Chen et al., 2002) in length, causing foam banks on shores during the bloom decline. Phaeocystis globosa is globally detected (Xu et al., 2003; Schoenemann et al., 2005; Medlin and Zingone, 2007) and in the North Atlantic is most abundant during May–June in the eastern part, including the Greater North Sea (Gieskes et al., 2007).
Phaeocystis globosa and P. pouchetti show higher growth rates in colonies than for single cells (Veldhuis et al., 2005). Spreading of colonies was reported by division or release of cells (Rousseau et al., 1994). Losses of Phaeocystis in the southern North Sea were mainly attributed to sedimentation (Riebesell, 1993). Flagellates and colonies are grazed by microzooplankton, such as ciliates and heterotrophic flagellates (Peperzak et al., 1998; Stelfox-Widdicombe et al., 2004) or by a wide array of vertebrates including fish (Weisse et al., 1994).
Phaeocystis can excrete up to 30% of its assimilated biomass (Peperzak et al., 2000a) and production of exopolymeric particles following nutrient limitation has been reported (Mari et al., 2005). Phaeocystis is a major producer of dimethylsulfoniopropionate (DMSP), an antioxidant (Sunda et al., 2002). DMSP is a precursor of dimethyl sulphide (DMS), which is an important global climate regulator. In the Belgian coastal zone, Phaeocystis accounted for 50% of annual primary production and 78% of DMS production (Gypens et al., 2014).
Phaeocystis species succeed the spring bloom of diatoms when silicate becomes limiting and irradiance has reached 100 Wh/m2per day (Peperzak et al., 1998), although they can also grow in parallel with diatom blooms before silicate becomes limiting. Phaeocystis is a good competitor under nitrogen-limitation (Riegman et al., 1992). Along Belgian, Dutch and German coastal waters Phaeocystis blooms have been observed frequently since about 1974 (Cadée and Hegeman, 2002), partly succeeded by the accumulation of foam on beaches (Hanslik and Rahmel, 1995) and sometimes dominating phytoplankton abundance (Raabe et al., 1997).
Various researchers have found a link between nutrient enrichment of marine waters and an increase in Phaeocystis blooms (e.g. Cadée and Hegeman, 1986; Lancelot et al., 1987; Brockmann et al., 1990), especially in coastal waters where the influence of nutrient input via rivers is highest.
Data for this assessment were only available for Belgian, Dutch and German coastal waters and have mainly been compiled from national data banks. These include: Landesamtes für Landwirtschaft, Umwelt und ländliche Räume (LLUR), Niedersächsischer Landesbetrieb für Wasserwirtschaft, Küsten- und Naturschutz (NLWKN), Alfred Wegner Institut (AWI), Rijkswaterstaat, (RWS), and the Management Unit of the North Sea Mathematical Models and Scheldt estuary (MUM). Helgoland data are protected by AWI, so data were obtained from Germany’s national OSPAR COMP assessment 2007 (OSPAR, 2008).
Owing to restrictions on the use of Helgoland AWI data, the analyses and results presented in this assessment are limited. Since 2005, data were available from fewer monitoring sites. International Council for the Exploration of the Sea (ICES) data for Phaeocystis pouchettii in the northern North Sea and the English Channel 1903–1911 were not considered.
Cell numbers have been estimated from fresh samples (2001–2004 only) or Lugol-fixed samples (since 2005 only). Parallel data were statistically significantly correlated (natural log scales). When colonial cells were not counted as loose colonial cells it was assumed that colonies contain 1000 cells each for calculating cell numbers. Sampling frequencies varied from weekly, to bi-weekly, to monthly, or only occasional.
Spatially, the south-eastern North Sea area was divided into squares of 716.5 km2, for which local average concentrations were calculated. Temporally, time series was divided into regular sections (months or annual bloom seasons: April–August) enabling calculation of averages and their variability, and confidence assessments (Brockmann and Topcu, 2014). By this method the different data resolutions are smoothed and bias towards sites with more frequent sampling is avoided. Spatial gradients as well as time series were calculated with the same software (Surfer, Golden Software). The R-tool was not used because it has been shown that results are identical with the Surfer tool.
Seasonal average and maximum abundance were analysed, taking into account sampling restrictions. In general, surface data are representative of the full water column because there is vertical mixing in the mostly shallow areas. At stratified locations, Phaeocystis was predominantly found in the upper mixed layer. Trends were analysed using the Mann-Kendall test.
Résultats
Cette évaluation utilise les données de la surveillance des phaeocystis de trois pays; la Belgique (1990–2009), les Pays-Bas (1990–2014) et l’Allemagne (2001–2014). Les sites de surveillance disposant de données sur les phaeocystis se situent dans les eaux côtières; ils sont principalement sur la côte et le long de certains transects perpendiculaires à la côte (Figure 1).
Dans tous les sites de surveillance néerlandais (1990–2010) et belges (1988–2009) et presque tous les sites de surveillance allemands (2003–2012), les teneurs en cellules de phaeocystis les plus élevées se produisent durant la saison de croissance, d’avril à mai. Dans la partie septentrionale de la côte allemande (LLUR, Figure 1) elles se produisent plus tard, en juin habituellement.
Les espèces de phaeocystis atteignent généralement leur maximum saisonnier en avril et mai, l’évaluation s’est donc focalisée sur les gradients spatiaux de l’abondance et des tendances temporelles des phaeocystis durant cette saison. On n’a relevé aucune tendance temporelle significative statistiquement dans les données analysées.
La Figure 2 montre la répartition spatiale des teneurs maximales en phaeocystiséchantillonnées durant le printemps et l’été (avril–août) de 1990 à 2014. L’abondance des phaeocystisau cours de cette période était élevée le long de la côte sud-est de la mer du Nord (côtes néerlandaise et allemande). Les abondances maximales révèlent les « points chauds » régionaux où les teneurs locales en cellules de phaeocystis peuvent dépasser 100 millions de cellules par litre.
Le profil spatial des teneurs en phaeocystis reflète la salinité moyenne dans la mer du Nord méridionale qui est influencée par les rejets fluviaux dans le cadre du courant côtier résiduel. On a également identifié des tendances spatiales semblables dans les teneurs en nutriments ( teneurs en nutriments ).
La disponibilité des données et la méthodologie inspirent une confiance modérée.
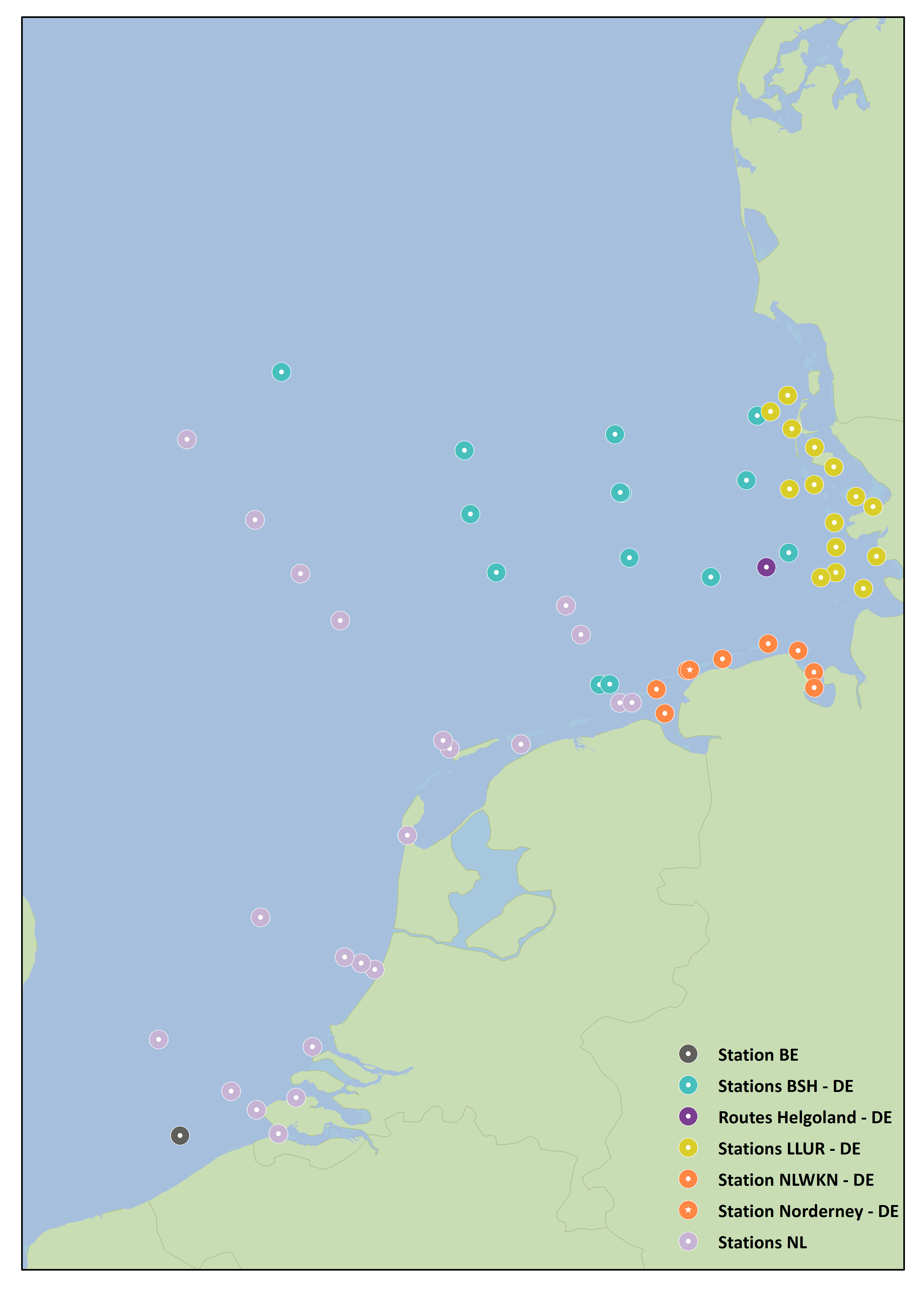
Figure 1: Sites de surveillance des phaeocystis par la Belgique (BE), les Pays-Bas (NL) et l’Allemagne (DE) (2003–2012)
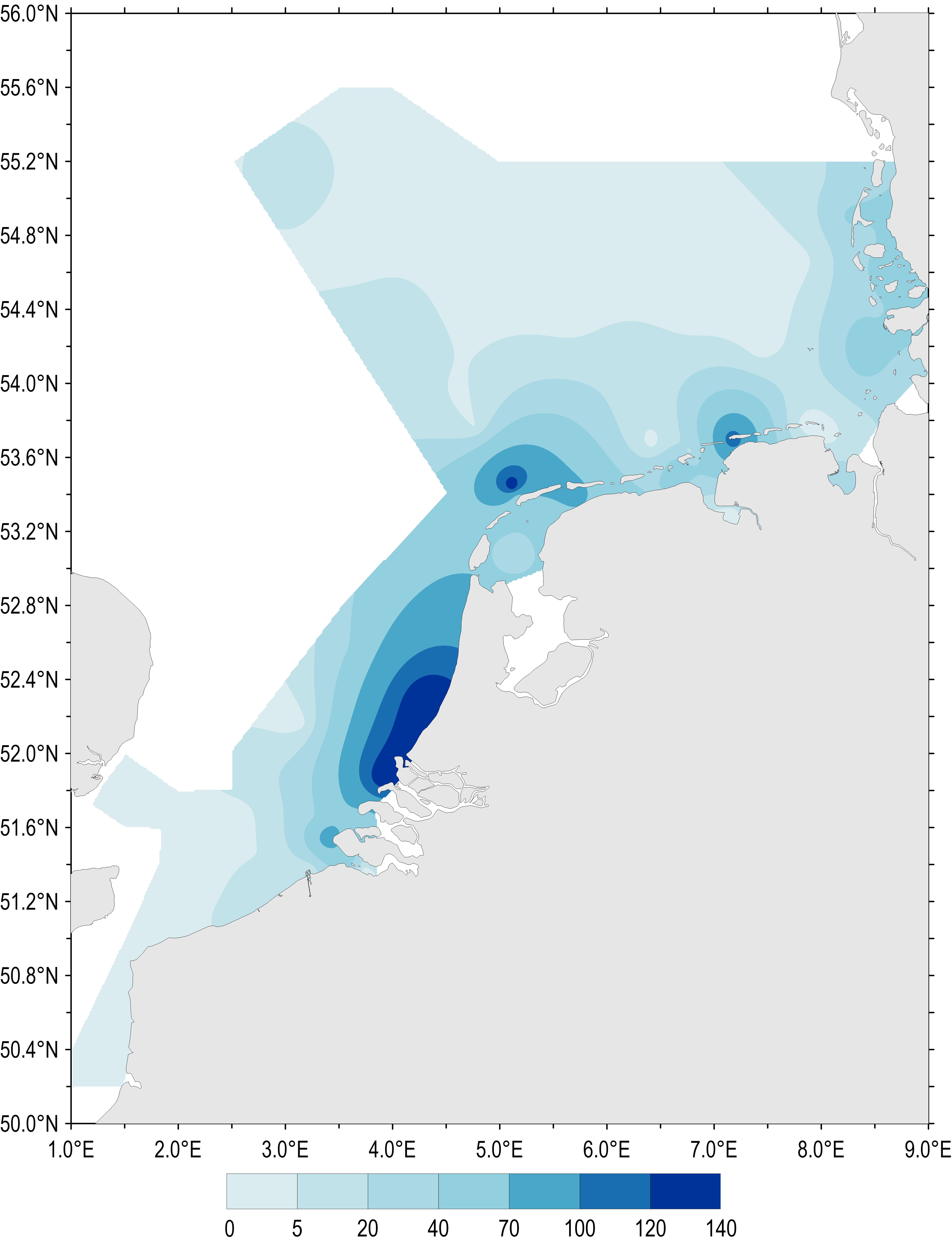
Figure 2: Gradients des teneurs maximales en phaeocystis (millions de cellules par litre) durant la période d’avril à août (toutes les années et sites de surveillance disponibles, 1990–2014)
The assessment focused on spatial gradients and temporal trends in Phaeocystis during the growing season, April–August (Figure b).
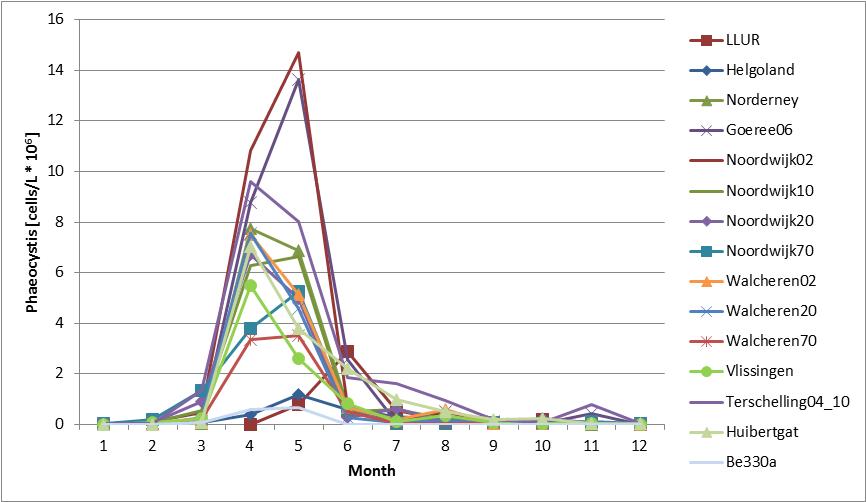
Figure b: Annual cycle of average abundance of Phaeocystis at Belgian (Be 330a; 1990–2009), Dutch (1990–2014) and German (Helgoland Reede, LLUR, Norderney, 2001–2014) stations
The Phaeocystis time series shows high spatial and temporal variability at and between monitoring sites (Figure candFigure d). The time series of average abundance showed cell numbers were particularly in 1991, 1997–1999 and 2008 (Figure c). At Helgoland and Norderney, high cell numbers (over one million cells per litre) were counted in more than 20 successive seasonal measurements. Locally, Phaeocystis cell numbers are variable with maximum abundance at Dutch monitoring sites during 1991, 1994 and 1998 (Figure d). At Helgoland, high cell numbers (over one million cells per litre) were counted during May and June 2001–2005 (except for 2003). Interannual fluctuations ranged from near zero to over 30 million cells per litre, with peaks of up to 100 million cells per litre for maximum values. The fluctuations were probably not caused by nutrient concentrations, which were much less variable (nutrient concentrations indicator assessment).
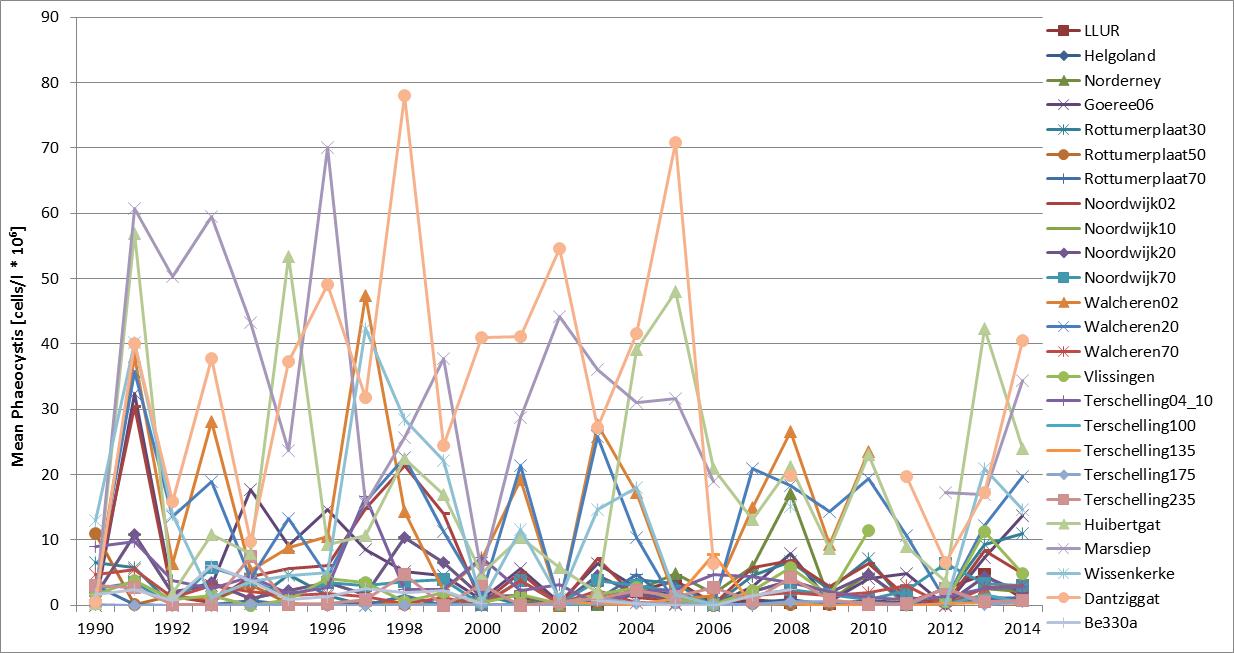
Figure c: Annual average abundance (April–August) of Phaeocystis at Belgian (Be 330a; 1990–2009), Dutch (1990–2014) and German (Helgoland Reede, LLUR, Norderney, 2001–2014) monitoring sites
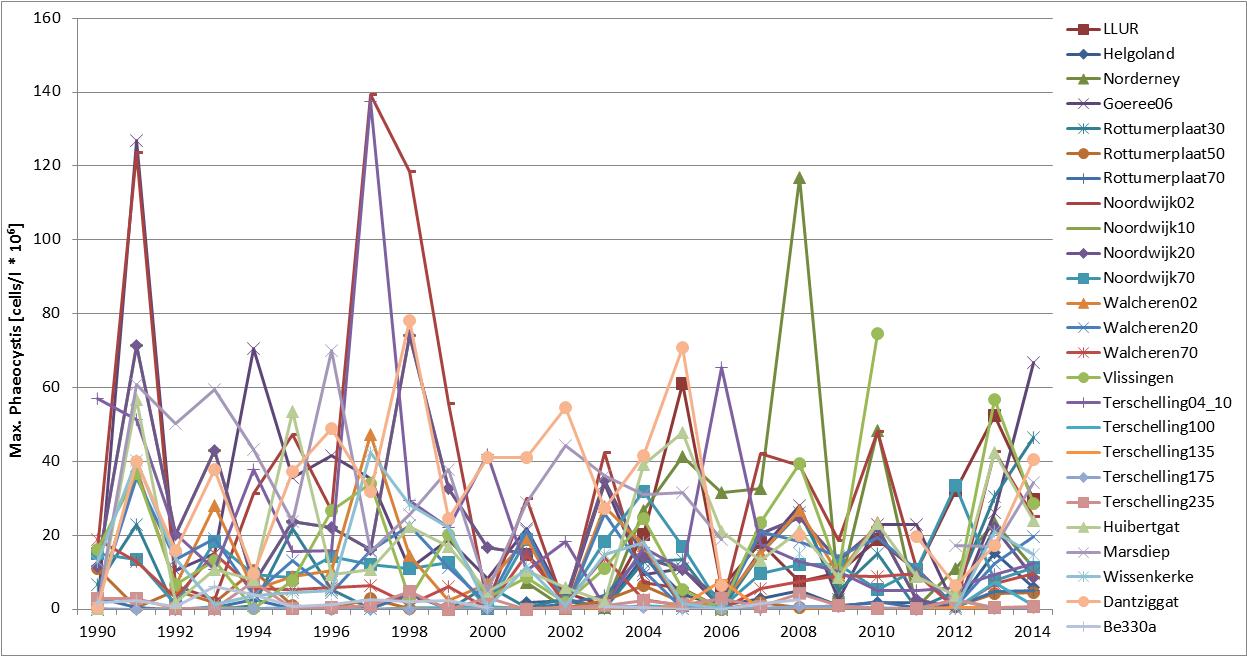
Figure d: Maximum abundance (April–August) of Phaeocystis at Belgian (Be 330a; 1990–2009), Dutch (1990–2014) and German (Helgoland Reede, LLUR, Norderney, 2001–2014) monitoring sites. For monitoring site locations see Figure 1
Samples obtained during the period April–May 1990–2014, were combined for testing the spatial distribution of seasonal Phaeocystis abundance (Figure 2 and Figure e). During the growing season average concentrations of Phaeocystis were highest at several close inshore locations along the Dutch and German coasts, 1990–2014 (Figure e).
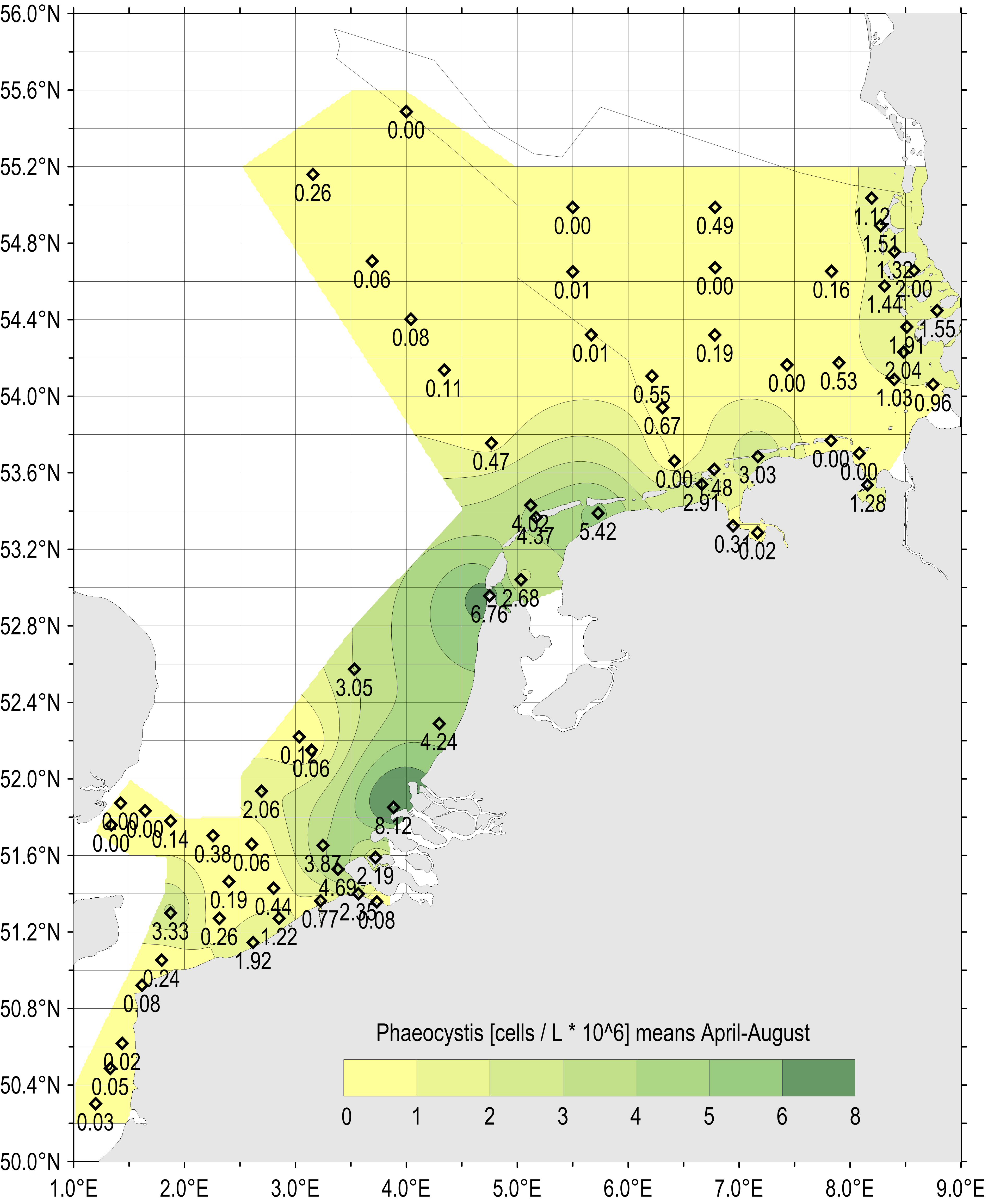
Figure e: Gradients of average Phaeocystis concentration for the period April–August (all available years and monitoring sites 1990–2014)
Spatial gradients of elevated Phaeocystis concentrations along the Dutch coast match the gradients measured at German monitoring sites. Separately, high average abundances were measured at one Belgian monitoring site in the English Channel and at the northern German coast (LLUR monitoring sites).
Although high nutrient inputs may lead to Phaeocystis blooms, annual average concentrations of Phaeocystis in the south-eastern North Sea were especially high (over one million cells per litre) before the 1960s and after the 1980s, periods when nutrient inputs from human activities were relatively low (Gieskes et al., 2007). In the Marsdiep 1990–2014, blooms of over one million cells per litre persisted for the longest periods (more than 100 days per year) around 1990 (Cadée and Hegeman, 2002).
Conclusion
Des teneurs élevées en phaeocystis, phytoplancton marin nocif, dans les eaux côtières (souvent supérieures à un million de cellules par litre et pouvant atteindre jusqu'à 100 millions de cellules par litre), ces teneurs diminuant offshore, peuvent se produire en réaction à des teneurs élevées en nutriments et pourraient indiquer une eutrophisation.
Cette évaluation montre que les efflorescences de phaeocystis sont maximales durant la période de croissance estivale (avril–juin) dans la mer du Nord méridionale. La taille des efflorescences varie grandement d’une année a l’autre, les teneurs moyennes saisonnières pouvant aller de presque zéro a plus de cinq millions de cellules par litre. Ces fluctuations sont probablement dues à une combinaison de divers facteurs, tels que la luminosité, la température, la salinité et autres influences hydrodynamiques et la disponibilité des nutriments. Les teneurs en nutriments sont cependant plus cohérentes et moins variables que celles des phaeocystis dans la mer du Nord méridionale ( évaluation de l’indicateur des teneurs en nutriments ). De plus on n’a relevé aucune tendance temporelle ou spatiale significative statistiquement dans les données analysées.
The continuing high abundance of Phaeocystis (often over one million cells per litre, sometimes over 30 million cells per litre, and with peaks in maximum concentration of up to 100 million cells per litre) along the shallow continental coasts correlates with high nutrient concentrations and could be an indication of eutrophication. However, the factors driving the interannual fluctuations in concentration need more research, especially parallel sampling of potentially confounding parameters, such as nutrient ratios, changes in organic matter, stratification, vertical distribution of single cells and colonies, and residence time within the upper mixed layer.
The exact determination of Phaeocystis blooms as observed along the coasts of Belgium, the Netherlands and Germany is difficult owing to the occurrence of different ecotypes and live stages with different growth rates and grazing pressure. The specific indication of surplus nitrogen and phosphorus is limited because of the high interannual variability that is not related to the average nutrient regime. The development of colonies is not only affected by nitrate concentration but also by the light climate, temperature and grazing pressure. The assumed dependence on nutrient ratios (N/Si or P/Si) was not confirmed.
Allelopathic effects of Phaeocystis on other phytoplankton species (Hansen and Ellertsen, 2007) should be investigated more generally, including in relation to competition for nutrients. Conversely, mortality of Phaeocystis globosaby viruses has been reported (Baudox et al., 2006).
Lacunes des connaissances
Les données sur les phaeocystis disponibles pour l’évaluation se limitaient, sur le plan régional, à la mer du Nord méridionale. Il a été difficile de déterminer la durée des efflorescences, l’échantillonnage étant restreint. Des données récentes sur les phaeocystis n’ont pas encore été complètement notifiées et stockées au Conseil international pour l’exploration de la mer (CIEM). Il faudrait estimer les contributions des phaeocystisà la biomasse totale du phytoplancton pour pouvoir réaliser une évaluation représentative par rapport aux teneurs en chlorophylle-a.
Des recherches supplémentaires sont nécessaires pour identifier les raisons de la forte variabilité interannuelle des teneurs en cellules.
Several studies indicate that Phaeocystis abundance in the southern North Sea is to a largely extent climate-related, and determined by the amount of Atlantic Ocean water flushed through the English Channel and Dover Strait. From a management perspective it would be useful to know the extent to which that elevated Phaeocystis concentrations in this area are climate-related.
Further investigation is needed of the link between climate change and Phaeocystis abundance (Gieskes et al., 2007).
Baudox, A.C., A.A.M. Noordeloos, M.J.W. Veldhuis, C.P.D. Brussaard, 2006, Virally induced mortality of Phaeocystis globosaduring two spring blooms in temperate coastal waters. Aqu. Microb. Ecol. 44, 207-217
Brockmann, U., R.W.P.M. Laane, H. Postma, 1990, Cycling of nutrient elements in the North Sea. Neth. J. Sea Res. 26 (2-4), 239-264
Brockmann, U.H., D.H. Topcu, 2014, Confidence rating for eutrophication assessments. Mar. Poll. Bull. 82,127-136
Cadée, G.C. & J. Hegeman, 1986, Seasonal and annual variation in Phaeocystis pouchetii (Haptophyceae) in the westernmost inlet of the Wadden Sea during the 1973 to 1985 period. Neth. J. Sea Res. 20, 29-36
Cadée, G.C. & J. Hegeman, 2002, Phytoplankton in the Marsdiep at the end of the 20th century; 30 years monitoring biomass, primary production, and Phaeocystis blooms. J. Sea Res. 48, 97-110
Chen, Y.Q., N. Wang, P. Zhang, H. Zhou, L.H. Qu, 2002, Molecular evidence identifies bloom-forming Phaeocystis (Prymnesiophyta) from coastal waters of southeast China as Phaeocystis globosa. Biochem. System. Ecol. 30, 15-22
European Council (EC), 1991. Directive 91/676/EEC of 12 December 1991 concerning the protection of waters against pollution caused by nitrates from agricultural sources
ECJ 2009. European Court of Justice Judgment of the Court (Third Chamber) of 10 December 2009. European Commission v United Kingdom of Great Britain and Northern Ireland. Failure of a Member State to fulfil obligations – Environment – Directive 91/271/EEC – Urban waste water treatment - Article 3(1) and (2), Article 5(1) to (3) and (5) and Annexes I and II – Initial failure to identify sensitive areas – Concept of ‘eutrophication’ – Criteria – Burden of proof – Relevant date when considering the evidence – Implementation of collection obligations – Implementation of more stringent treatment of discharges into sensitive areas. Case C-390/07 European Court Reports 2009 I-00214
Gieskes, W.W.C., S.C. Leterme, H. Peletier, M. Edwards, P.C. Reid, 2007, Phaeocystis colony distribution in the North Atlantic Ocean since 1948, and interpretation of long-term changes in the Phaeocystis hotspot in the North Sea. Biogeochemistry 83, 49-60
Gypens, N., A.V. Borges, G. Speeckaert, C. Lancelot, 2014, The dimethylsulfide cycle in the eutrophied southern North Sea: a model study integrating phytoplankton and bacterial processes. PLOS ONE 9, e85862, 15pp.
Hansen, E. & H.C. Ellertsen, 2007, Do the polyunsaturated aldehydes produced by Phaeocystis pouchetii(Hariot) Lagerheim influence diatom growth during the spring bloom in northern Norway? J. Plankton Res. 29, 87-96
Hanslik, M. & J. Rahmel, 1995, Seasonal dynamics of bloom-forming and toxic algae along the coast of Lower Saxony since 1982. DHZ 5, Suppl., 221-235
Lancelot, C., G. Billen, A. Sournia, T. Weiße, F. Colijn, M.J.W. Veldhuis, A. Davies, P. Wassmann, 1987, Phaeocystis blooms and nutrient enrichment in the Continental coastal zones of the North Sea. Ambio 16 (1), 38-46
Mari, X., F. Rassoulzadegan, C.P.D. Brussaard, P. Wassmann, 2005, Dynamics of transparent exopolymeric particles, TEP) production by Phaeocystis globosa under N- or P-limitation: a controlling factor of the retention/export balance. Harmful Algae 4, 895-914
Medlin, L. & A. Zingone, 2007, A taxonomic review of the genus Phaeocystis. Biogeochemistry 83, 3-18
OSPAR, 2008, Second OSPAR integrated report on the eutrophication status of the OSPAR maritime area. OSPAR, London, 372/2008. 107pp.
OSPAR 2010. The North-East Atlantic Environment Strategy. Strategy of the OSPAR Commission for the Protection of the Marine Environment of the North-East Atlantic 2010–2020 Agreement 2010-3
OSPAR 2013. Common Procedure for the Identification of the Eutrophication Status of the OSPAR Maritime Area Agreement 2013-08
Peperzak, L., F. Colijn, W.W.C. Gieskes, J.C.H. Peeters, 1998, Development of the diatom-Phaeocystis spring bloom in the Dutch coastal zone of the North Sea: the silicon depletion versus dayly irradiance threshold hypothesis. J. Plankton Res. 20, 517-537
Peperzak, L., R.N.M. Duin, F. Colijn, W.W.C. Gieskes, 2000a, Growth and mortality of flagellates and non-flagellate cells of Phaeocystis globosa(Prymnesiophyceae, J. Plankton Res. 22, 107-119
Peperzak, L., F. Colijn, E.G. Vrieling, W.W.C. Gieskes, J.C.H. Peeters, 2000b, Observations of flagellates in colonies of Phaecystis globosa (Prymnesiophyceae); a hypothesis for their position in the life cycle. J. Plankton Res. 22, 2181-2203
Raabe, T., U.H. Brockmann, C.D. Dürselen, M. Krause, H.J.Rick, 1997, Nutrient and plankton dynamics during a spring drift experiment in the German Bight. Mar. Ecol. Prog. Ser. 156, 275-288
Riebesell, U., 1993, Aggregation of Phaeocystis during phytoplankton spring blooms in the southern North Sea. Mar. Ecol. Progr. Ser. 96, 281-289
Riegman, R., A.A.M. Noordeloos, G.C. Cadee, 1992, Phaeocystis blooms and eutrophication of the continental zones of the North Sea. Mar. Biol. 112, 479-484
Rousseau, V., D. Vaulot, R. Casotti, V. Cariou, J. Lenz, J. Gunkel, M. Baumann, 1994, The life cycle of Phaeocystis (Prymnesiophyceae, evidence and hypotheses. J. Mar. Systems 5, 23-39
Schoenemann, V., S. Becquevort, J. Stefels, V. Rousseau, C. Lancelot, 2005, Phaeocystis blooms in the global ocean and their controlling mechanisms: A review. J. Sea Res. 53, 43-66
Stelfox-Widdicombe, C.E., S.D. Archer, P.H. Burkill, J. Stefels, 2004, Microzooplankton grazing in Phaeocystis and diatom-dominated waters in the southern North Sea in spring. J. Sea Res. 51, 37-51
Sunda, W., D.J. Kieber, R.P.Kiene, S. Huntsman, 2002, An antioxidant function for DMSP and DMS in marine algae. Nature 418, 317-320
Veldhuis, M.J.W., C.P.D. Brussaard, A.A.M. Noordeloos, 2005, Living in a Phaeocystis colony: a way to be successful algae species. Harmful Algae 4, 841-858
Weisse, T., K. Tande, P. Verity, F. Hansen, W. Gieskes, 1994, The trophic significance of Phaeocystis blooms. J. Mar. Systems 5, 67-79
Xu, N., Y. Qi, J. Chen, W. Huang, S. Lv, Y. Wang, 2003, Analysis of the cause of Phaeocystis globosaScherffel red tide. Acta Sci. Circumst. Huanjing, 23, 113-118