Impacts on the provision of ecosystem services by marine food webs
Given the current changes occurring in marine food webs, no positive outcome for the ecosystem services they provide are observed or expected, except that research and education activities will probably increase due to the generally unknown status of food webs. In addition to climate change, fishing pressures, physical disturbance, nutrient inputs, introduction of non-indigenous species and underwater noise can all impact food web structure and functioning. Adverse changes in marine food web structure, richness and functioning can affect the provision of ecosystem services by species including all trophic levels in marine ecosystems.
This section evaluates the impact that changes in the environmental state of food webs, as described in the ‘ State ’ section, have on the ecosystem services that the North-East Atlantic provides. The section was developed on the basis of a literature review combined with expert judgement, using the same methodology across all thematic assessments. Several workshops involving ecosystem services experts and food-web experts were held to discuss and agree the presented results. A detailed description of the anthropogenic pressures that impact food-web state, and thus the provision of ecosystem services, is provided in the ‘ Pressures ’ section. The following section provides further details of the role that food webs (and their state) play in relation to the provision of the ecosystem services outlined in Figure I.1.
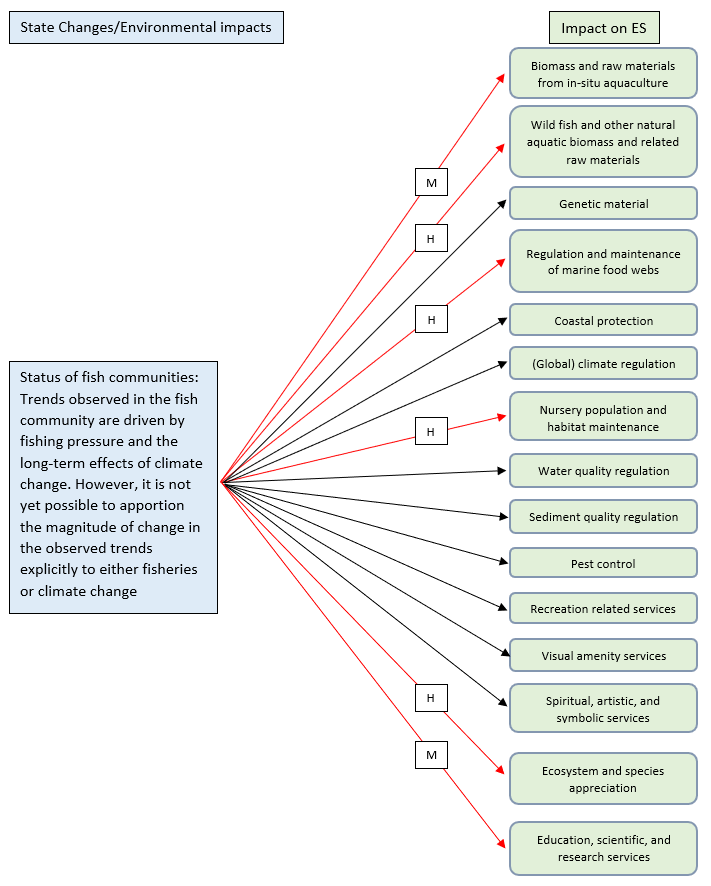
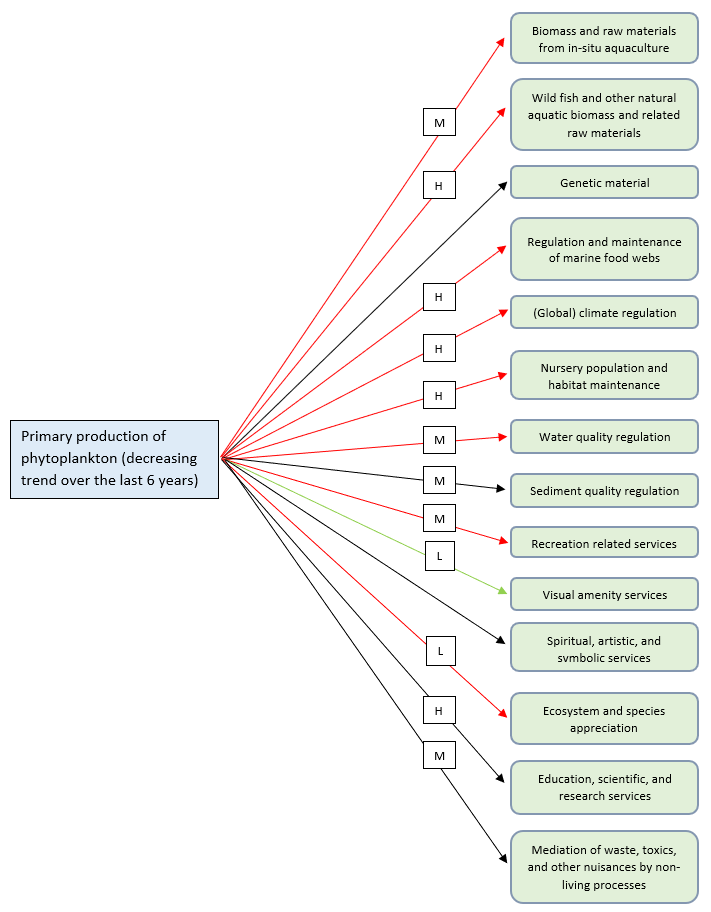
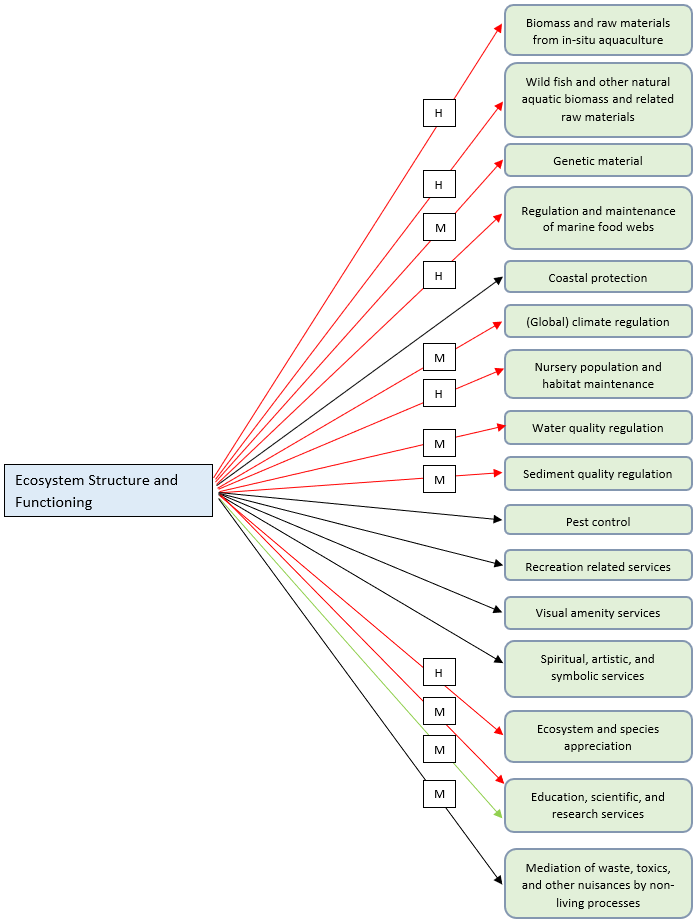
Figure I.1: Schematics depicting the 'State (changes)’ - ‘Impacts on ES’ linkages for the Food Webs thematic assessment, using three trophic guild state changes as the starting point. The ecosystem services shown are those considered most relevant in relation to the Food Webs thematic assessment. Each arrow also denotes an expert estimate of the nature and magnitude of the impact (black arrow = recognition of the existence of the impact but no clear negative or positive impact (uncertain nature); red arrow = negative impact; green arrow = positive impact; H = high impact; M = medium impact; L = low impact; U = unknown impact)
Detailed rationale for the role that food webs (and their state) play in relation to the provision of ecosystem services
Marine food webs are made up of and sustained by all the organisms that make up marine ecosystems. Accordingly the various trophic guilds, be they marine mammals, marine invertebrates, fish, phytoplankton, zooplankton and others, play differing and important roles in sustaining the dynamics of marine food webs. The following section provides further details of the ecosystem services associated with food webs and their state.
The original concept of Mean Trophic Level (Common Indicator Assessment on Changes in Average Trophic Level of Marine Consumers , FW4) was known as "fishing down marine food webs". A decline in the Middle Trophic Level of a fishery indicates a shift from intense fishing pressure on higher trophic levels to increasing fishing pressure on lower trophic levels, due to a decline in the biomass of higher trophic levels; this can have cascading consequences for marine food webs and consequently for the ecosystem services supported by their balanced functioning (Essington et al., 2006; OSPAR, 2017h). Fishing mortality also limits the age structure of fish communities, reducing the proportion of older and larger individuals. In addition to the average trophic level of marine predators, the indicators Proportion of Large Fish (Large Fish Index) (FC2), Candidate Indicator Pilot Assessment of Mean Maximum Length of Fish (FC3) and Size Composition in Fish Communities (FW3) are used to monitor the impacts of fishing mortality and, by providing information on the status of fish communities they also provide useful information on the status of marine food webs.
It has been shown that declines in predatory fish generate significant increases in their prey, considerably modifying the interactions between higher trophic levels and potentially inducing cascading effects on lower levels, including decreases in zooplankton and increases in phytoplankton (Eriksson et al., 2011). For example, decreases in the biomass of predators in offshore areas can lead to increases in the abundance of mesopredators along the coast. Coastal mesopredators can regulate the abundance of crustacean and gastropod herbivores (mesograzers), with consequent effects on marine vegetation. Accordingly, it has been observed how an increase in coastal mesopredators, leading to a decrease in important mesograzers and in combination with nutrient growth due to coastal eutrophication, can lead to declines in beds of eelgrass (Eriksson et al., 2011). In addition, there is the example of the central Baltic Sea, where in the past a mesopredator increase following a decrease of their predators (cod) led to a trophic cascade, including a 50% decrease in zooplankton and a doubling in phytoplankton biomass. This can lead to an increase of carbon fixation associated with increased primary productivity ((Global) climate regulation) (Eriksson et al., 2011; Holmlund and Hammer, 1999). In the context of OSPAR IA 2017, an increase in the biomass of marine predators was observed. An increase in the availability of predators in the marine ecosystem can reduce the vulnerability of the ecosystem to natural or human-induced pressures (OSPAR, 2017h). However, a significant increase in predators could lead to a decrease in zooplanktivorous predators, and thus to an increase in zooplankton, leading in turn to a decrease in phytoplankton and the associated primary productivity.
The food-web cascading effects could locally and negatively affect the ecosystem service of ‘(global) climate regulation’. Similar reasoning can be applied to marine predators in general (whales, large pelagic fish, sea birds, pinnipeds, etc.) which, through food web interactions, have been shown to be essential in supporting the provision of ecosystem services by coastal plant communities including ‘coastal protection’, ‘(global) climate regulation’, ‘habitat maintenance’ and the balance of the food web itself (Atwood and Hammill, 2018). Thus, it is evident that changes in the balance of food webs can have cascading effects leading to shifts in the structure of food webs and consequently to alterations in the provision of ecosystem services underpinned by different organisms.
To add to the relationship between the chosen indicators and the food web dynamics, large fish can contribute to food web maintenance and the enhancement of primary productivity via nutrient transport (Tavares et al., 2019). In this regard, fish species with a large body size that are particularly sensitive to the additional mortality associated with contribute effectively to the maintenance of food webs (OSPAR, 2017h; Tavares et al., 2019). It has been observed that decreases in fish body size due to environmental pressures and impacts associated with human activities can have negative consequences for traits such as egg production and nutrient transport, and in turn for ecosystem services such as ‘regulation and maintenance of marine food webs’ and ‘wild fish and other natural aquatic biomass and related raw materials’ (Oke et al., 2020; Tavares et al., 2019). In a similar way, a decline in the abundance of such species can negatively affect food webs (Tavares et al., 2019).
In the context of the present work, few direct links were found in the existing scientific literature between the individual state indicators and the provision of specific ecosystem services. It follows that, links to the ecosystem services that are in most cases cited as being provided by the dynamics of marine food webs can be assumed. In fact, state changes such as an alteration in the proportion of large fish (FC2) and depletion of the mean maximum length of fish (FC3)), combined with greater scarcity of individual fish and species, can lead to altered food-web structures (Tavares et al., 2019). As shown by the Candidate Indicator Pilot Assessment of Feeding Guilds (FW7), large fish are typically piscivorous, so their depletion could be expected to lead to an increase in their prey within the planktivore and benthivore guilds, with potential ramifications for pelagic and benthic habitats.
Primary production of phytoplankton:
Marine phytoplankton is a well-known provider of ecosystem services, crucially contributing to the climate cycle and functions of the marine ecosystem and beyond. Phytoplankton is one of the main marine ecosystem components that contributes most to providing the ecosystem service of ‘(global) climate regulation’. Some phytoplankton species produce dimethylsulfoniopropionate (DMSP), a precursor to dimethyl sulphide (DMS), important to the process of cloud formation. Phytoplankton is also a major contributor to the carbon fixation process and its sequestration in sediments and ocean depths, by capturing CO2 from the atmosphere (Tweddle et al., 2018). At the same time, as a food source for pelagic herbivores (including larval stages), phytoplankton also forms the basis of most marine food webs (‘regulation and maintenance of marine food webs’), supporting the production and supply of biomass from the upper trophic levels, including biomass produced in the marine aquaculture or mariculture contexts (wild fish and other natural aquatic biomass and related raw materials and biomass and raw materials from in-situ aquaculture). Spatial variability in primary productivity and phytoplankton abundance can influence the spatial distributions of upper trophic levels, including fish, birds, and marine mammals, and indirectly the local provision of ecosystem services by these organisms (See: Marine Birds Thematic Assessment , Marine Mammals Thematic Assessment , and Fish Thematic Assessment for more details about these ecosystem services). It can therefore be argued that the functioning and balance of food webs underpinned by the primary production of phytoplankton has the capacity to indirectly support the provision of ecosystem services by all those marine organisms that are part of the food web itself. For this reason, it can also be argued that the maintenance of balanced food webs can positively contribute to the provision of all the marine ecosystem services considered in the QSR 2023, including ‘pest control’ and all ‘cultural ecosystem services’.
As confirmation, in relation to this specific thematic assessment, visual links to several ecosystem services (e.g. cultural ecosystem services) have been provided, even if all the ecosystem services have not been individually addressed in the narrative. This is due to the overall importance of balanced food web functioning in ensuring the good status of marine ecosystems and thus their provision of ecosystem services. All the ecosystem service mechanisms mentioned in Marine Birds Thematic Assessment , Marine Mammals Thematic Assessment , Fish Thematic Assessment , Benthic Habitats Thematic Assessment , Pelagic Habitats Thematic Assessment flow into this thematic assessment and vice versa, because all marine ecosystem components contribute to food webs.
Shelf areas support most oceanic primary production and they are at the same time the areas affected by most human activities and related pressures (e.g. oil and gas, wind energy, wave and tidal renewable energy, aquaculture, aggregate dredging and waste dumping; Tweddle et al., 2018). However, the Candidate Indicator Pilot Assessment of Primary Productivity (FW2) showed several site-specific changes that do not permit a generalised conclusion for the whole OSPAR Maritime Area. This means that areas where a downward trend in annual phytoplankton production was observed, the provision of associated ecosystem services (described above) is likely to decrease. The opposite can be said for areas where high phytoplankton production can lead to an increase in the local provision of ecosystem services such as support for biomass production from shellfish farming activities (OSPAR, 2017h). In the current assessment cycle, primary production is decreasing in many assessment units. Given the general trend for the OSPAR Maritime Area, the impact of the current state of phytoplankton primary production in the OSPAR area on the related ecosystem services is mainly represented with red arrows (Figure I.1), indicating a negative impact on most of those services. Nevertheless, some of the relationships between the impact on ecosystem services and primary production remained unclear, represented by black arrows (Figure I.1). Declining primary production is likely to increase water clarity which, in turn, may have a positive effect on visual ecosystem services. This relationship is represented by the green arrow (Figure I.1).
Despite the overall trend of decreasing primary production, the role played by anthropogenic pressures and their impacts in relation to food webs and components such as phytoplankton must be kept in mind, as they nevertheless act in the background. For example, phytoplankton is essential in the benthic-pelagic coupling that is crucial to the functioning and balance of food webs, as it involves functions from nutrient cycling to energy transfer between habitats (Griffiths et al., 2017). At the same time, anthropogenic pressures and related environmental impacts (addressed in the pressure-related thematic assessments) influence benthic-pelagic coupling through their impacts on the physical and biological components of the marine ecosystem, including phytoplankton. For example, a climate change-related increase in water temperature may cause changes in the timing, and decrease the magnitude, of phytoplankton blooms, which in turn may lead to a decrease in the transport of organic matter to the benthos and reduce the release of inorganic nutrients from the marine sediment. Meyer at al., (2018) found changes in macrofauna community structure in the south-eastern North Sea caused by increasing sea surface temperature and de-eutrophication effects between 1986, 2000, 2010 and 2015. De-eutrophication effects in line with food limitation caused by decreasing phytoplankton biomass and pelagic and benthic primary production strengthen the dominance of opportunistic species such as Phoronis spp. and Spiophanes bombxy (Meyer et al., 2018). Overfishing can impact, for example, oyster reefs, resulting in a reduction in the water filtration processes which in turn triggers an increase in phytoplankton production and a reduction in water quality and clarity (Griffiths et al., 2017). These components of the marine ecosystem, as a result of environmental impacts from human-related pressure, can alter the structure and dynamics of food webs and consequently the provision of ecosystem services.
Changes in phytoplankton communities and biomass and changes in zooplankton communities and abundance:
Phytoplankton communities are directly linked to zooplankton communities. The presence and abundance of the zooplankton influences the production of biomass at higher trophic levels (‘wild fish’ and other ‘natural aquatic biomass’ and related ‘raw materials’) and of the biomass in maricultural contexts (‘biomass and raw materials from in situ aquaculture’). The contribution of zooplankton in sustaining the balance of the food web itself (‘regulation and maintenance’ of marine food webs) is also detectable in its role as a grazer for algae and bacteria and as a provider of nitrogen and phosphorus (‘nutrient cycling’) that has positive effects on the primary productivity of phytoplankton. In addition, zooplankton, through processes such as the export of particles by grazing, the fractioning of sinking particles and the transport of particulate organic carbon at depth through its diel vertical migration, plays a crucial role in the functioning of the oceanic biological carbon pump that contributes to the ecosystem service of regulation of atmospheric CO2 levels (‘(Global) climate regulation’) (Lomartire et al., 2021). Changes in the ratio of planktonic life forms, as well as changes in their absolute and relative abundance, can be used to detect changes in community functioning, which in turn can inform changes in ecosystem services (Bedford et al., 2020; McQuatters-Gollop et al., 2019).
OSPAR assessments have shown that over the most recent period (since 2000) zooplankton abundance has tended to decrease while phytoplankton biomass has tended to increase (OSPAR, 2017i). In addition, OSPAR assessments in studies of planktonic life forms have shown that plankton communities (phytoplankton and zooplankton) have experienced significant changes, indicating alterations in ecosystem functioning (OSPAR, 2017i). However, although these assessments show that important changes have occurred, they are preliminary, and it is difficult to draw conclusions about extent and direction, and what the consequences are for overall marine ecosystem dynamics and the provision of ecosystem services in the OSPAR Maritime Area. For this reason, the links between the trends observed for phytoplankton (biomass and primary production) and zooplankton (abundance) and ecosystem services are represented as links that exist but whose nature cannot yet be clearly defined as overall positive or negative for the OSPAR area (black arrows Figure I.1). However, by presenting and describing the ecosystem services underpinned by phytoplankton and zooplankton it is possible to at least identify which of the ecosystem services may be altered as a result of major changes in phytoplankton biomass and productivity and zooplankton abundance and community functioning (Bedford et al., 2020).
The above-mentioned structural changes in the different ecosystem components of a food web due to climate change and anthropogenic pressures are linked to changes in the trophic structure and functioning of food webs. For example, food webs at higher temperatures will become less organized and shift towards detritus-based food webs (Baird et al., 2019). Results have shown, inter alia, an intensification of detrital production and consumption, a substantial increase in total system throughput, a decline in consumption of phytoplankton and macrophytes, and an increase in community respiration (Baird et al., 2019). Non-indigenous species influence the production and biomass of wild animals, which in turn changes ENA indices and food web system attributes. ENA indices show declines in the number of trophic cycles and in trophic efficiency, ascribed to the impact of non-indigenous species on system organization and function (Baird et al., 2012; Jung et al., 2020).
As the supply of ecosystem services depends on the functioning of ecosystems and the ecological groups composing them, using Ecological Network Analysis ( Candidate Indicator Pilot Assessment of Ecological Network Analysis Indices , FW9) to quantify the provisioning of ecosystem services can provide multiple benefits. It considers the interconnectivity of marine and coastal ecosystems to adequately forecast the effects of ecosystem changes, it can quantify simple ecological functions, like secondary production, as well as more complex ones, and it can assess a wide range of ecosystem services owing to their holistic and integrative nature (Nogues et al., 2022). For this reason, in Nogues et al. (under review), ENA was used to quantify the potential effects of climate change on species distribution and the reef and reserve effects of an offshore wind farm on the provision of ecosystem services. Multiple ecosystem services were considered, such as ‘food supply’ from fisheries (through catches), ‘cultural benefits’ from the production of flagship species such as marine mammals and seabirds, and the ability of the system to withstand disturbance through redundant trophic pathways (relative redundancy). The use of ENA enabled the observation of connections between ecosystem services. As the offshore wind farm increased habitat heterogeneity, the biomass of multiple benthic and demersal species increased, with a beneficial effect for catches, but also for culturally important species such as dolphins and porpoises. This was due to the increased amount of potential prey available to these high trophic level groups within the offshore wind farm, leading to higher production of these iconic species (Nogues et al., under review). This demonstrates how useful ENA could be in the context of ecosystem services quantification.
State | Response |