Impacts of marine climate change on biodiversity and human activities
The changes in physical and chemical conditions in the marine environment are causing changes in the state of the marine ecosystem and in the human activities taking place in the marine environment. Changes in state have been reported from across the OSPAR Maritime Area and have been identified across the three non-climate themes in NEAES 2030: clean seas, biologically diverse and healthy seas and productive and sustainably used seas. In Arctic Waters (Region I), changes are occurring at an accelerated rate, with changes in state affecting many aspects including pelagic habitats, marine mammals, and a range of human activities. Low-lying areas, particularly those surrounding the Greater North Sea (Region II) are at greater risk of impact from rising sea levels. The impacts of climate change on biodiversity and human activities are now widespread across the OSPAR Maritime Area. Although, for some, the processes and attribution are only tentatively described (often due to a relatively short time series or lack of understanding of the complex processes and interactions between cumulative pressures), there is a growing body of evidence that these impacts are caused by climate change. As the climate continues to change, further impacts are likely to emerge, as well as become progressively more severe.
The physical and chemical pressures produced by climate change are affecting the state of the marine ecosystem and its habitats, and of the human activities taking place, across the OSPAR Maritime Area. The following paragraphs outline the changes in state linked to climate change for the three remaining themes in NEAES 2030.
Clean seas
There is limited evidence of direct causal changes to levels of hazardous substances, marine litter, eutrophication or radioactive substances due to climate change and ocean acidification. However, the links between them are generally well identified. Increasingly, there is good evidence that there are co-benefits to be derived from climate action alongside direct interventions on source contaminants. The climate impacts on these four parameters can be placed in the following three categories: (1) changes in the toxicology of contaminants at warmer temperatures; (2) changes in the pathways of contaminants; and (3) changes in the frequency of weather conditions that lead to episodic inputs of contaminants from land and rivers and to the remobilisation of historic pollutants. In particular, the episodic inputs and remobilisation may originate from changing precipitation patterns, including more extreme precipitation events, resulting in increased run-off. These could lead to greater inputs of litter, hazardous substances, nutrients and radioactive substances from the terrestrial environment. Changes in oceanic transport due to climate change could then further affect the distribution of these inputs across the OSPAR Maritime Area.
Marine litter
The Marine Litter Thematic Assessment identifies a number of ways in which climate change could affect the quantities of marine litter entering the marine environment, especially from rivers, and then the subsequent distribution and deposition of the litter. While there may be a need to adapt to some of the changes, it is not currently a major factor influencing OSPAR’s marine litter objectives or the selection of actions for OSPAR’s Regional Action Plan on Marine Litter.
The exact effects that climate change will have on marine litter pollution in the North-East Atlantic are relatively unstudied and still difficult to predict. Therefore, the potential effects described within that Thematic Assessment are generally hypothetical and highly uncertain, but form a starting point for further exploration of the issue. In general, climate change will not directly affect marine litter or its impact on biota per se, but its influence on atmospheric and ocean circulation may affect some of the pathways for and retention of litter. By managing marine litter, the cumulative impacts on marine biota may also be reduced, thus supporting increased resilience to climate change.
Hazardous substances
There is substantial evidence that increases in temperature and extreme events may enhance the release, transportation, and mobilisation of both hydrophobic and hydrophilic pollutants in the marine environment. Also, the toxicity of pollutants may increase with increasing temperatures. Climate change also contributes to oxygen depletion (hypoxia and anoxia) in the marine environment, which can increase the uptake of methylmercury in food webs and in several cases has also been found to increase the toxicity of contaminants. Changes involving extreme storms and waves may also increase the likelihood of accidents, including those where hazardous substances are released into the marine environment (see: Offshore Industry Thematic Assessment ).
The risks of climate change-induced increases in pressures and in contaminant concentration levels are reducing the impact of the responses made to reduce inputs, particularly those from the historic contaminants stored in sediments, and could be a contributing factor in the lack of progress made towards a better status for the effects of hazardous substances in the marine environment. Further work on attributing the changes in contaminant concentrations to ocean climate changes may be needed in order to understand and address this aspect.
Radioactive substances
There is little evidence that climate change is having any impact on radioactive substances within the OSPAR Maritime Area at present. Increased levels of naturally occurring radionuclides in the Arctic Ocean have been linked to a reduction in the depth of permafrost and increased mobility of these radionuclides in soils (Kipp et al., 2018). However, there is potential for predicted climate change effects (IPCC, 2021) to influence many aspects of radioactive substances in the marine environment, and this could have an impact on the assessments currently being carried out by OSPAR, as well as on the underlying parameters of such assessments. Warming seas may also affect the uptake of radionuclides by marine biota and food web structures, leading to changes in the biological transfer of radionuclides.
Climate change may have the potential to affect the sources of radioactive substances in the OSPAR Maritime Area, owing to predicted increasing sea levels and storm surge events leading to increased remobilisation of radionuclides from coastal sediments, as well as threatening the safety of coastal nuclear facilities.
The potential contribution of nuclear technologies in mitigating climate change is discussed in the Response Section . Some Contracting Parties consider that nuclear fission and, potentially, nuclear fusion have a role to play in fulfilling this need and have developed national policies that incorporate the use of nuclear energy to further their decarbonisation objectives.
Eutrophication
The Eutrophication Thematic Assessment does not highlight climate change as a primary cause of current changes in eutrophication status, but does note that the effects of eutrophication are increased by climate change. This co-occurrence derives from the activities (e.g. burning of fossil fuels, agriculture, deforestation) that contribute to climate change, as well as the associated changes in prevailing climatic conditions (pressures) that are linked to eutrophication (e.g. changes in rainfall and run-off, storms, stratification, ocean acidification).
Climate change may also have an impact on levels of nutrient enrichment, in that it could lead to changes in circulation patterns and the occurrence and duration of stratification, which could then impact nutrient levels and primary production. Expectations about the direction of the effects are uncertain (Holt et al., 2016, Schrum et al., 2016).
Increased nutrient loading could be expected if river discharges increased, but this also depends to a large extent on future land use and socio-economic developments (Arheimer et al., 2012, Bartosova et al., 2019).
Climate change may also have an impact on the direct and indirect effects of nutrient enrichment. Increased water temperatures have been shown to lead to phenological shifts, biogeographical changes and changes in abundance of plankton (see Brander et al., 2016, for an overview). With changes in phytoplankton composition, changes in chlorophyll concentrations and primary production can be expected.
Climate change can impact upon dissolved oxygen concentration in many ways, most evidently via the direct effect on solubility. However, it can also increase metabolic rates and oxygen demand and increase stratification, which inhibits the supply of oxygenated waters to lower levels. The duration of seasonal stratification in shelf seas is expected to increase and regions that show oxygen depletion are expected to become larger (Wakelin et al., 2020).
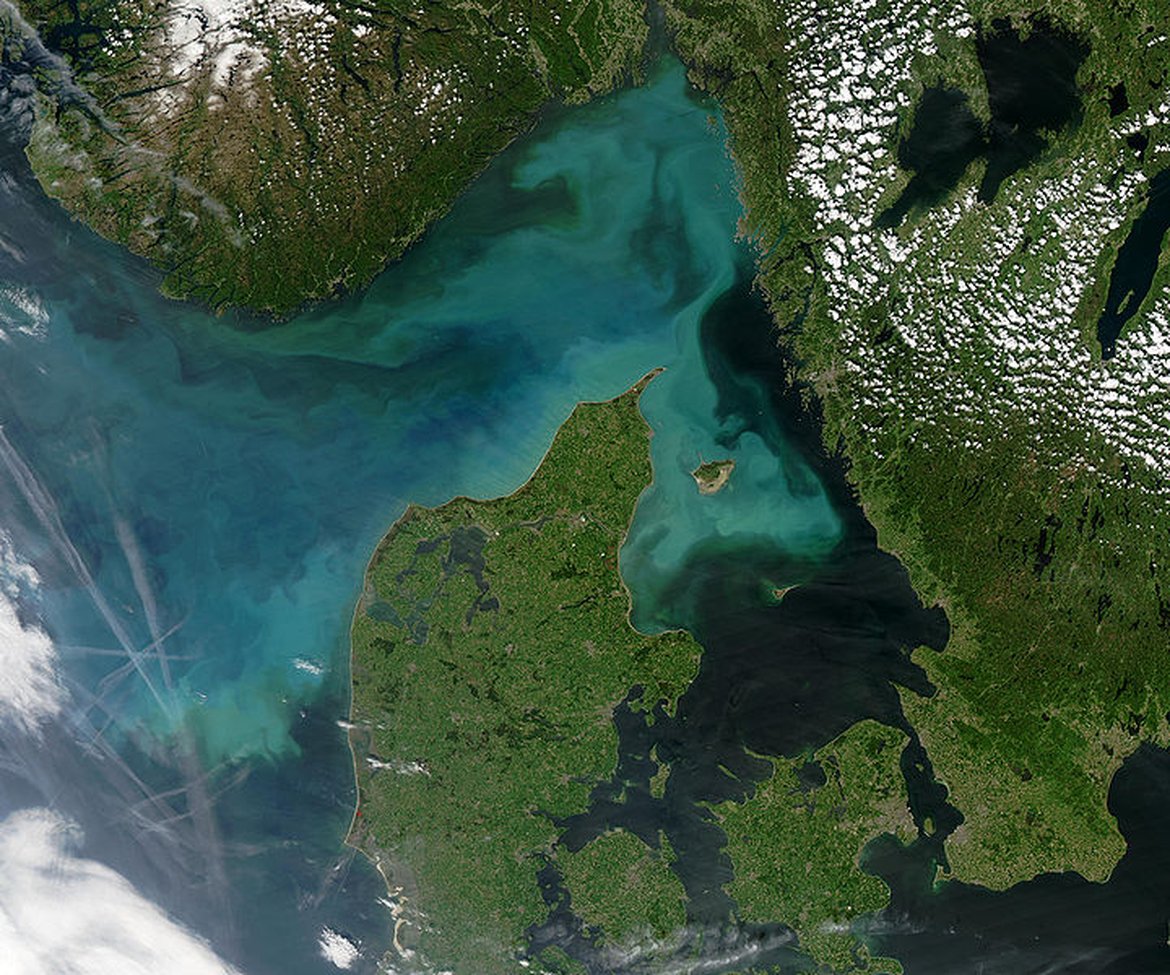
NASA Satellite imagery of bloom in Skaggerak and Kattegat Seas © NASA
Biologically diverse and healthy seas
There is increasing evidence of the impacts of climate change on the marine ecosystem, its function and biodiversity. These can generally be categorised as: (1) habitat loss; (2) shifts in distribution; (3) changes to species composition and food webs; and (4) changes to life history events.
Habitat loss
Changes in environmental conditions driven by climate change are likely to have impacts on marine biodiversity by influencing the availability of suitable habitats.
Mammals and birds
Sea-level rise and extreme weather events are the main climate-related factors impacting mammals and birds. Sea-level rise leads to increased mortality of juvenile mammals. Mammals breeding along low-lying coastal areas are particularly vulnerable to storm surges (Evans & Bjorge, 2013; Zicos et al., 2018), with reported extremes of up to 75% of grey seal pups being lost after severe storms (SCOS, 2018). Additionally, reduced area and ice condition may impact seals through loss of birthing habitat and of refuge habitat from predators such as killer whales (ICES, 2018; ICES, 2021; ICES, 2022; Marine Mammals Thematic Assessment ). Increased storm frequency plays an important role in waterbirds’ nest survival and the survival of adults trying to forage during storms. In addition, climate change may affect the extent and quality of waterbirds’ breeding and wintering grounds as well as their migration stopovers. However, short-term extreme weather events (i.e. storms) are, to date, less impactful on seabird population size than climate-mediated changes in prey availability (Johnston et al., 2021). On the other hand, there is growing evidence that short term extreme weather events will become more frequent and have increasingly significant effect (Mitchell et al., 2020; Marine Birds Thematic Assessment ).
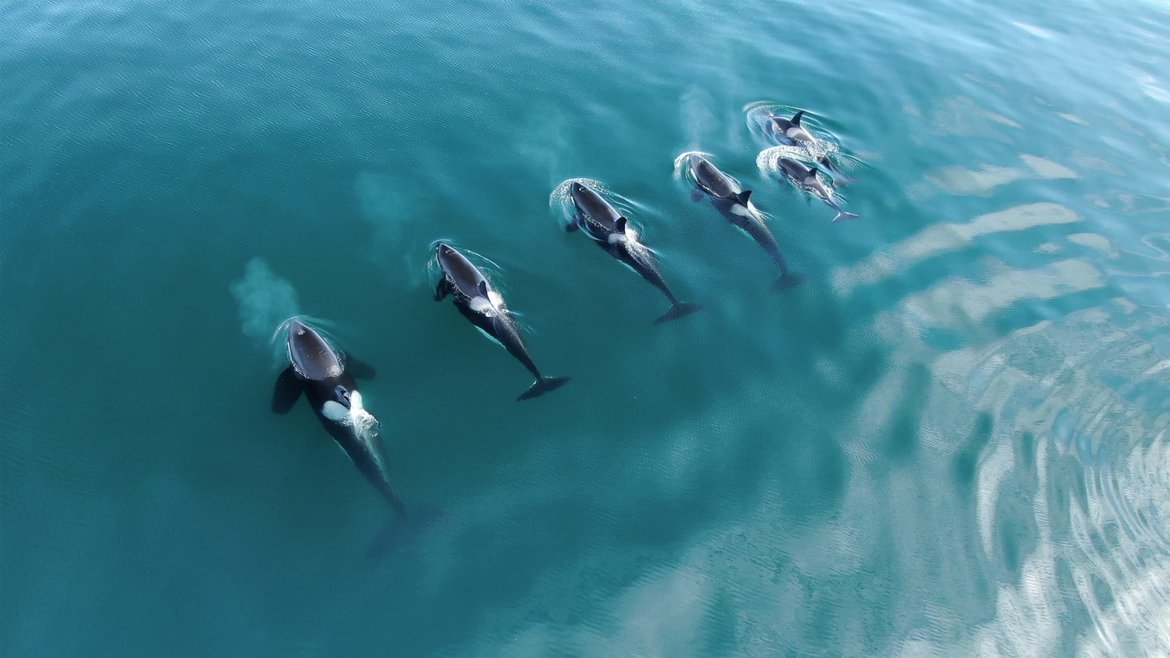
Sea-level rise and extreme weather events are the main climate-related factors impacting mammals such as orcas. © Shutterstock
Fish
Temperature, the main factor impacting the suitability of fish habitats, can affect fish in different ways. For cod and haddock, earlier spawning in warmer years at the northern edge of their distributional range increases their survival during the subsequent winter months (Ottersen, 2000). On the other hand, Arctic and boreal fish are predicted to be particularly impacted (Poloczanska et al., 2013) by warming climate, and the wolffish Anarhichas lupus has been declining in abundance (Bluemel et al., 2022). The observations are more contradictory for herring: according to Lyashevska et al. (2020), herring might be more susceptible to climate warming at the southern limit of the distribution range, compared with northerly populations. By contrast, recruitment, body size and spawning stock biomass for Norwegian spring-spawning herring have been found to be positively correlated with temperature (Graham & Harrod, 2009). In addition, increasing bottom water temperatures have caused a deepening response among some fish species (Dulvy et al., 2008) and the availability of suitable habitat at increasing depth may limit species range shifts under future climate scenarios (Rutterford et al., 2015; Fish Thematic Assessment ).
Benthos
Ocean warming, acidification, changes in oxygen fluxes, increased water turbidity/reduced light penetration and sediment resuspension have been reported to impact all benthic habitats, habitat suitability for sensitive benthic species, community structures and diversity patterns (Harley et al., 2006; Hoegh-Guldberg & Bruno 2010; Poloczanska et al., 2013; Gattuso et al., 2015; Nagelkerken & Connell, 2015; Poloczanska et al., 2016; Weinert et al., 2016). However, each habitat is susceptible to different main impacts (Krause-Jensen et al., 2021).
Increased sea temperature and changes in coastal development, in terms of coastal protection due to sea level rise, are expected to have a particularly strong effect on coastal areas such as the littoral zone, infralittoral rocks, biogenic reefs, Zostera beds and mud flats (Birchenough et al., 2015, Garrard & Tyler-Walters 2020). These habitats are unable to migrate landwards and are particularly threatened by increasing water depths, which leads to risks of habitat loss and coastal squeeze through impacts such as oxygen depletion caused by nutrient enrichment (Painting et al., 2013).
Extreme events involving sea level and altered hydrological and sediment dynamics along the shoreline affect benthic biodiversity (Spreybroeck et al., 2006). This may be further increased by storms and storm surges which translocate and disperse large sediment volumes, thus altering sediment properties and reducing habitat heterogeneity. The impact on benthic habitats will increase with the magnitude, frequency and spatial scale of storm events (Corte et al., 2017). On the other hand, sedimentary habitats are liable to adapt to a gradual sea level rise and compensate for alterations by extra sedimentation. Stratification effects may cause changes to deep sea benthic habitats, though the impacts here are unclear (Sweetman et al., 2017). Acidification can impact the integrity of coral reefs and cause ecosystem-scale habitat loss (Hennige et al., 2020; Benthic Habitats Thematic Assessment ).
Pelagic
The impacts of increasing temperature, acidification and light attenuation increase on the abundance of plankton lifeforms have been most frequently detected in shelf and oceanic pelagic habitats. In the North-East Atlantic, the ocean climate-related stressors with the greatest impact on plankton communities are sea surface temperature warming (e.g. Harris et al., 2014, Costoya et al., 2015) and ocean acidification (e.g. Beaugrand et al., 2013). Furthermore, climate can alter wind speed and precipitation, potentially affecting the light attenuation coefficient (Cappuzzo et al., 2015) and sea surface salinity (Holt et al., 2010). The abundances of dinoflagellates and small and large copepods have been found to decline across the North-East Atlantic, while meroplankton abundance has been observed to increase throughout the Greater North Sea. Although meroplankton in the coastal and shelf areas of the Greater North Sea have increased in abundance, simultaneously the abundance of holoplankton is declining in the shelf regions (see: Pelagic Habitats Thematic Assessment ). Observations of North Atlantic krill show a squeeze in habitat due to a northward shift in warm isotherms that is not matched by movement of the polar front (Edwards et al., 2021).
Food webs
Depletion of the territory and ice conditions under which seals give birth and whales avoid predators, the greatest fear being killer whales, will lead to major changes in food webs (ICES, 2018; ICES, 2021; ICES, 2022).
Understanding the extent and magnitude of all the different impacts is important, because they could result in reductions of sensitive habitats and species as well as continued declines in biodiversity.
Distribution shift
Increasing evidence indicates that geographic shifts of marine biodiversity towards northern ranges are largely driven by changes in sea surface temperature and associated changes in primary production, as species adjust to changes in environmental conditions and in the abundance and distribution of prey (ICES, 2016). These may be categorised as tropicalization (Horta e Costa et al., 2014), meridionalization (Punzón et al., 2016) and borealization (Fossheim et al., 2015). The high latitude regions have become increasingly dominated by species with warmer affinities (ICES, 2008; Lenoir et al., 2014; Simpson et al., 2011). Climate change impacts are expected to be more pronounced in Region I, where species are more restricted in their ability to adapt and move further north (see: Marine Mammals Thematic Assessment and Fish Thematic Assessment ).
Pelagic
Latitudinal shifts have been observed in a wide range of pelagic species, particularly the zooplankton taxa. For example, range shifts of Calanus zooplankton taxa have been observed, with Calanus helgolandicus (associated with warmer water habitat) replacing Calanus finmarchicus (associated with colder waters) (Beaugrand, 2003) (see: Pelagic Habitat Thematic Assessment ).
Birds
Temperature-mediated shifts in distribution and earlier departures of seabirds (change in timing of life events) have been observed within the OSPAR Maritime Area, with some species becoming increasingly concentrated in north-eastern areas during winter and fewer reaching south-western parts of their former range (so-called “short-stopping”). The effects are often species- and region-specific, thus contributing to the difficulties in attributing broad-scale changes (see: Birds Thematic Assessment ).
Marine mammals
Shifts in the range of some cetacean species have been observed (see: Marine Mammal Thematic Assessment ). For example, Williamson et al. (2021) found a northward shift in the occurrence of strandings of warm-water adapted species (short-beaked common and striped dolphins) related to changes in sea-surface temperature, in OSPAR Regions II and III.
Fish
Recent assessments of the long-term distributional shifts of key commercial fish stocks in Europe have found distributional shifts for all examined species (Baudron et al., 2020; ICES, 2016). Species identified as ‘big movers’ include anchovy, white anglerfish, cod, megrim, haddock, hake and plaice (ICES, 2016). Increases in abundance and biomass are predicted for fish stocks in high latitude regions (Arctic and subarctic) and the poleward tips of the continental shelf margin, while declines are anticipated within the tropics and at the southern margins of semi-enclosed bodies of water (e.g. the Celtic Sea and the Bay of Biscay; Cheung et al., 2010) (see: Fish Thematic Assessment ).
Benthos and NIS
The effect of seawater warming is related to expectations of a general northward expansion of marine species (Pinsky et al., 2013; Poloczanska et al., 2016), with some evidence and indications of climate-related changes affecting increasing abundances of non-indigenous species (NIS) in marine systems (García-Gómez et al., 2020; Sorte et al., 2010; Staehr et al., 2020). The northernmost regions of the North Atlantic are predicted to experience the greatest impacts under worst-case climate scenario predictions. Increased temperature and lower pH will lead to declines in population densities, loss of biodiversity and reduced biogeographic distribution that might compromise connectivity across large time scales as well as long-term survival. Nowadays, warm-water benthic taxa are more common in the Barents Sea (ICES, 2021; Jørgensen et al., 2022). Considering the different pathways of NIS introduction, a northward expansion seems most relevant in the case of secondary introductions, as NIS introduction hotspots in southern seas would introduce new NIS to northerly regions where temperature conditions gradually became favourable. Secondary introductions (~spread) have, however, accounted for only 5% of the NIS introductions in the OSPAR Regions. Overall, there is currently no strong evidence of climate change-related northward spread of NIS.
Climate change influences marine species composition and food-webs
Changes in species composition driven by climate change have been observed for marine organisms of every habitat, triggering further changes in trophic interactions. As the base of the food web, the changing phytoplankton and zooplankton communities have consequent effects on the benthic and pelagic organisms which rely on them for food, and in turn on their top predators, such as seabirds and marine mammals. These changes often take place on a local or regional scale and their characteristics differ between the OSPAR Regions (Baird et al., 2019).
Phytoplankton are highly sensitive to changes in physical and chemical conditions, namely temperature, salinity, light, nutrients, pH, storminess and currents, as well as to the availability of oxygen and CO2. Each of these factors is influenced by changing climate (see: Pressures section ). Changes in ice-associated biota communities are strongly linked to the thinning and reduction of sea ice cover in OSPAR Region I (AMAP, 2017).
More generally, changes in prey species within marine food webs may result in distribution range shifts of the mobile predator species which follow them, or may have effects on predator population density and size as prey becomes less valuable or available, leading to lower species performance, e.g. for example breeding failure or reduced biomass (for further details and examples see the Food Webs Thematic Assessment ).
Primary productivity from phytoplankton may be augmented by nutrient enrichment due to climate change-induced increased riverine input after extreme rainfall events, and, in colder regions, from loss of sea ice. However, other human impacts may interact with these impacts, and there may also be increased potential for harmful algal blooms as a result of climate change. Decreasing sea surface salinity impacts zooplankton diversity in shelf habitats of the Celtic Seas (OSPAR Region III), but also in oceanic habitats of the Bay of Biscay and the Iberian coast (OSPAR Region IV). While changes in plankton communities (encompassing lifeforms, biomass, abundance and diversity) may also be influenced by anthropogenic factors, climate change – which is associated with changing light attenuation, increasing temperature and sea surface salinity and their combined influence on stratification – has a strong impact on plankton communities. The effects of ocean acidification may then be an added stress factor. In all areas, predator-prey decoupling – between primary and secondary production – is expected to cause increased sinking of phytoplankton to the sea bottom (Morrison et al., 2019) (for further details and examples see the Pelagic Habitats Thematic Assessment ).
Like the pelagic ecosystem, benthic communities will be affected by climate change, resulting in changing species composition and predator-prey decoupling. In shallow water or coastal areas, sea-level rise, storminess and extreme weather events may change the community structure. The impacts on deep sea benthic habitats remain unclear: stratification may hinder the input of nutrients and, as with seasonal shelf sea stratification, productivity may be limited in summer. Winter storms may promote water-column mixing in winter (for further details and examples see Benthic Habitats Thematic Assessment ).
Warming could create environmental conditions that help introduced species to become established and spread, especially northwards (cf. Occhipinti-Ambrogi, 2021; Townhill et al., 2017; Poloczanska et al., 2016; Dukes & Mooney, 1999). While the NIS introduction rate is probably underestimated, higher NIS arrivals have been observed on southern European coasts (Tsiamis et al., 2019). The expected increases in biomass and abundance of NIS will change food web structure and functioning in all OSPAR Regions (Jung et al., 2020). However, there is only limited scientific documentation of causal effects from climate change on NIS (for further details and examples see Non Indigenous Species Thematic Assessment ).
The climate change impacts on seabirds include increased foraging difficulty due to extreme weather, changes in prey availability, increased predation and/or competition, increased heat stress on adults/chicks/eggs, and in some cases nest destruction caused by extreme climate events (see: Marine Birds Thematic Assessment ). As an example, lower over-winter survival and lower breeding success have been observed in black-legged kittiwake (Frederiksen et al., 2004, 2005, 2007; Frederiksen, 2014). This is due to the species’ dependence on sand eels, which in turn depend on copepod prey that have seen a northward shift at the time of peak abundance due to increased sea surface temperature. As sand eels are an important food for many seabirds, similar effects are expected to be observed for other species. In addition, extreme events are expected to have more impact in the future (Mitchell et al., 2020). Changes in food supply are evaluated as the major threat to seabirds by seabird conservation practitioners (Hakkinen et al., 2022). This changing relationship is also valid for other prey species like herring (Durant et al., 2003) and sprat (Österblom et al., 2006). Changing food availability not only impacts birds, but also marine mammals. Minke whales, for example, are observed to have declined near Iceland, probably because of lower food availability and a decrease in preferred prey species (Víkingsson et al.,2015; ICES, 2018).
The consequences described above, such as reduced or different prey availability, breeding failure due to habitat changes and changes in size and biomass, are also changes of concern for fishes (see: Fish Thematic Assessment ) and marine mammals (see: Marine Mammals Thematic Assessment ) in all OSPAR Regions.
(Eco)physiological alterations and changes in life history within species
As described above, altered timing in plankton growth may result in mismatches between prey and predators that are especially relevant for juvenile fish and their development. Increased physiological energy demand due to increased water temperature coincident with lower food supply – both due to climate change – may also explain reductions in fish biomass (Lotze et al., 2019). As juvenile fish are likely to grow more rapidly due to the ecophysiological effects of temperature, adult maximum sizes are expected to be reduced for metabolic reasons and adaptive life history traits (Genner et al., 2010, Ikpewe et al., 2021; see also Fish Thematic Assessment ). Climate change-induced higher water temperatures will also raise the metabolic demands of omnivorous zooplankton (Capuzzo et al., 2017; Thorpe et al., 2022; see also Pelagic Habitats Thematic Assessment ). Additionally, physiological heat intolerance is predicted to create regions that are unsuitable for species, causing local extinction in specific regions. For example, Arctic skua are expected to become less abundant in the British Isles (moderate confidence) and may even become entirely absent (low confidence; Pearce-Higgins et al., 2021; see also Marine Birds Thematic Assessment ). For top predators like sea birds and marine mammals, the melting of the Arctic ice also generates leakage from reservoirs of persistent chemicals, which bioaccumulate in the food web and interact with physiological processes (see: Marine Mammals Thematic Assessment ).
Other linkages
The reductions in sea ice cover in the Arctic due to climate change may exacerbate other human pressures on the ecosystem. These include increased disturbance from cargo vessels, expansion of wild capture fisheries into the region, and other industrial effects. These activities may cause further disturbance to Arctic mammal species and could lead to the introduction of NIS. Other cumulative impacts could be increased collision risk and by-catch and additional exposure to underwater noise.
Productive and sustainably used seas
Change in productivity or resilience of the ecosystem
The stress from climate change might exacerbate pressures from human activities and may further weaken ecosystem resilience. The changes will affect physical, chemical and biological cycles and may affect the ocean’s ability to stabilise the climate.
Stock distributions and/or sizes of fish and other seafood groups may change, for example through increased catch potential in high latitude areas (although impacts on commercially important stocks are uncertain) and decreases further south. The assessment, allocation, and transboundary management of catches may become increasingly complex and contentious. Fishers may need to adapt their practices, for example to address changes in the frequency of extreme events or to ensure resilience of fish stocks.
Climate change may also cause loss of aquacultural production or infrastructure due to increased intensity or frequency of extreme weather events. Diseases, harmful blooms, parasites and other negative effects on fish and shellfish production might increase due to physical/chemical changes in the environment. There will also be an increased risk of microbial pathogens and non-native species spreading from aquaculture systems into the environment and becoming established, if climate change creates more favourable conditions for them. The potential expansion of this sector into large-scale inshore and offshore facilities can potentially magnify some of the above issues. However, there is some possibility of positive impacts such as higher growth rates in certain warm-water finfish or shellfish aquaculture.
Change in ability to conduct the activity
Climate change has already led to other environmental changes such as increased frequency of storm events or the reduction in Arctic sea-ice coverage. These alterations will intensify in the coming years and alter the way human activities can be conducted.
Reduced ice coverage in the Arctic Waters Region could lead to further increases in shipping activity in some parts. How significant this will be remains uncertain, and depends on factors such as the length of the Arctic shipping season and the economic viability of trans-Arctic routes. Reduced ice cover and thus increased access may also result in the expansion of the offshore oil and gas industry in the Arctic, see Human Activities Thematic Assessment .
Changes in rainfall, extreme weather events, sea-level rise, increasing risk of marine pathogens and beach erosion could all affect marine and coastal tourism. Any associated recreational activities such as boating, coastal walking and water sports may also experience increased exposure to extreme, unsafe conditions (see: Human Activities Thematic Assessment ).
Change of the activity/pressure due to climate change
Several human activities are experiencing changes due to climate change, often altering their pressure footprint on the marine environment. These changes are likely to continue and, in some cases, accelerate.
Sea-level rise will increase the demand for sand for coastal reinforcement and maintenance, and thus increase aggregates extraction. It will also have an effect on the dredging activities which help to maintain shipping routes and facilitate harbour access, see Human Activities Thematic Assessment .
Extreme wind and wave events, shifting seafloor sediments and rising sea levels also present a changing hazard in the long term to offshore assets such as oil and gas installations, renewable energy infrastructure and subsea pipelines and cables. The vulnerabilities that can result from extreme storms and high wave events include the destabilization, scouring or degradation of offshore and subsea structures, health and safety risks to staff, reduced operating periods and reduced access for maintenance and inspection activities, thereby increasing the likelihood of accidents, including spill and pollution incidents (see: Human Activities Thematic Assessment and Offshore Industry Thematic Assessment ).
The expected large-scale development of offshore renewable energy capacity over coming decades may have impacts on local wind, wave, tide and sediment dynamics. There may be impacts on marine life, particularly on benthic environments surrounding the structures. To date, it is unclear how the expansion of offshore renewable energy, particularly wind energy structures, will interact with the impacts from climate change. This will depend on the region, time frame and scale of each development (see: Human Activities Thematic Assessment ).
Environmental changes in the physical properties of the ocean will influence levels of ambient underwater noise and the propagation of sound underwater. Climate-driven shifts in some activities, such as the relocation of shipping routes and fishing grounds and the expansion of offshore renewable energy, together with other potential effects from changes to, for example, vessel specifications and fishing fleet size and coverage will, in line with decarbonisation policies, result in different levels of anthropogenic underwater noise in the future. Activity restrictions, noise abatement and other mitigation measures may become more widely used to limit underwater noise levels (Faulkner et al., 2018), although these measures are likely to be focused on sensitive habitats/species and therefore be localised in their effect (see: Underwater Noise Thematic Assessment ).
Changing rainfall patterns and the impacts from extreme rainfall events will affect hydrological connectivity and the mobilisation of sediments, nutrients and contaminants (including microplastics) from agricultural land to waterways and in turn to marine waters. Sewer networks could become overloaded, leading to surface water flooding and overflow at urban waste water treatment plants, with untreated sewage along with litter flowing into waterways and/or coastal areas (see: Human Activities Thematic Assessment ).
There is also evidence of climate-related increases in the occurrence and spread of non-indigenous species in marine systems. The greatest risk here is predicted for the northernmost regions of the North Atlantic. Changes to shipping routes, for example as year-round trans-Arctic routes emerge, coupled with warming temperatures, will increase the risk of non-indigenous species being introduced through ballast water exchange and transport on ships’ hulls. Similarly, offshore man-made structures may act as stepping-stones for non-native invasive marine species (see: Non Indigenous Species Thematic Assessment ).
Pressures | Impact |