Eutrophication impact on ecosystem services
Eutrophication has a substantial impact, limiting access to ecosystem services by acting as a pressure on biodiversity and the ecosystem. Even at a low level, increased nutrient loads and changing proportions of nutrients result in biomass and phytoplankton species shifts which affect higher species. Species shifts are frequently characterised by bloom events which have significant economic impacts, as they reduce the attractiveness and amenity value of coastal waters. Increased phytoplankton biomass reduces light penetration, which in turn causes habitat loss by limiting areas where seaweeds and seagrasses can grow. These habitats are important for maintaining nursery populations. Healthy seaweeds and seagrasses themselves perform important water quality regulation functions, sequestering nutrients and binding sediment. More serious eutrophication involves hypoxic events which harm many organisms but are particularly damaging to sessile benthic fauna, whose loss again affects the food web and biotic water quality regulation. Extreme hypoxia and anoxia lead to a loss of both biotic and abiotic water quality regulation, as previously sequestered nutrients are lost from sediment surfaces and bacterial denitrification processes change.
This application of the Common Procedure identifies eutrophication problems in river plumes and coastal waters. These waters are under the most pressure, but also have the highest value to society in terms of recreation, visual amenity and artistic uses. These are the shallower areas where seagrasses and seaweeds would be expected to maintain nursery populations and habitat structure. They are also the recipients of land-based pollution, and the restoration of biotic and abiotic water quality regulation will be essential if eutrophication is to be addressed.
Qualification of impacts on ecosystem services
On the basis of the Eutrophication thematic assessment and expert judgement on how this relates to the ecosystem services associated with pelagic and benthic habitats, an estimate of the magnitude and direction of state change impacts on the relevant ecosystem services was made.
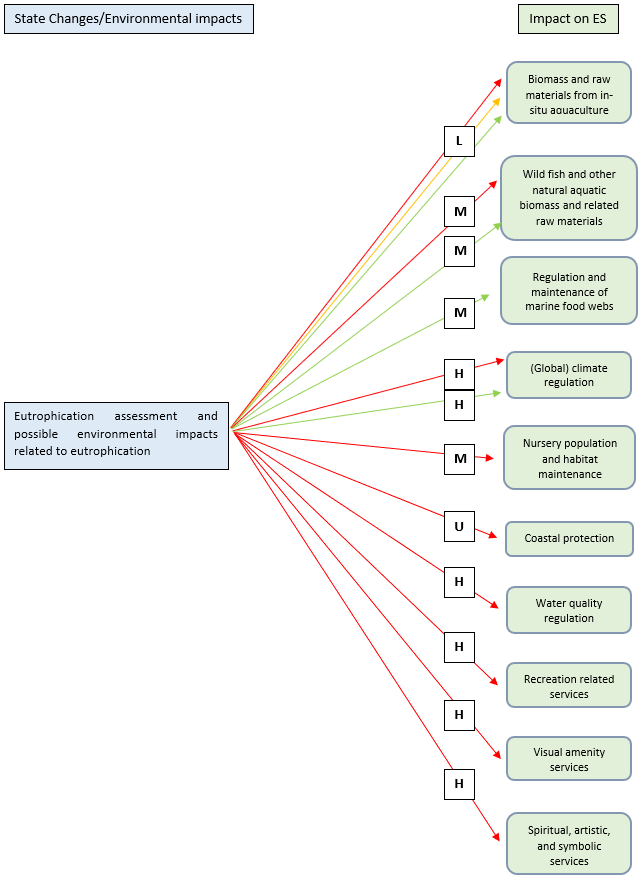
Figure I.1: Eutrophication assessment and possible impacts on ecosystem services related to eutrophication. H = high impact, M = medium impact, L = low impact, U = unknown impact. Arrow colours: green = positive, red = negative and yellow = neutral impact
Detailed rationale behind identified impacts on ecosystem services
Eutrophication is the result of excessive enrichment of water by nutrients. Eutrophication leads to increased growth of algae (phytoplankton) and plants, changes in the balance of organisms and degradation of water quality. The decomposition of algal and plant material by microbes promotes increased oxygen consumption in bottom waters, leading to hypoxia (a reduction of oxygen in the water) and subsequent deterioration of marine ecosystems and loss of biodiversity (EEA, 2015; Limburg et al.,2020; OSPAR, 2017k). Increasing levels of nutrients in the water, increased chlorophyll, reduced dissolved oxygen and increased occurrence of nuisance species associated with eutrophication lead to changes in the state of the environment through increased mortality, behavioural changes and health problems in marine organisms (e.g. suffocation due to lack of oxygen), reduced water quality, changes in the supply and quality of food for marine organisms, reduction in resources, and species shifts (OSPAR HASEC, 2021a). These environmental impacts are also associated with effects on benthos, increase in low-oxygen tolerant species, reduction in species diversity, changes in grazing capacity of species and changes in food web structure (OSPAR HASEC, 2021a). The environmental problems created by eutrophication are known to reduce the quality of ecosystem services (EEA, 2015).
Nuisance species can negatively impact those ecosystem services based on recreation and visual attraction and the associated psychological and mental well-being, through beach fouling and water- quality degradation. For example, increases in the local presence of microalgae and related chlorophyll concentrations reduce water transparency (O'Higgins and Gilbert, 2014). In the North Sea, eutrophication induces algal blooms along the coasts. Disruption to the plankton community is a phenomenon thought to be related to eutrophication affecting marine food webs and is known to have knock-on effects on aquatic biomass and consequently on fisheries. Foam deposits on beaches related to these bloom events also contribute to impacts on cultural ecosystem services associated with coastal environments (EEA, 2015). These events impact the provision of ecosystem services through loss of biodiversity, damage to the biomass produced in aquaculture settings (biomass and raw materials from in situ aquaculture), human harm (sea foam leading to the deaths of five surfers in 2020) and reduced attractiveness, thus affecting recreation and other cultural ecosystem services (EEA, 2015).
According to O'Higgins and Gilbert (2014), the main ecosystem services involved when considering eutrophication are the maintenance of balanced food webs (‘regulation and maintenance of marine food webs’), the service of habitat maintenance (nursery population and habitat maintenance), provision of aquatic biomass (wild fish and other natural aquatic biomass and related raw materials), climate regulation ((global) climate regulation), and services related to amenity and recreation values (visual amenity services and recreation-related services).
Algae, seagrasses and dissolved oxygen all play a role in habitat maintenance. In turn, the nursery population and habitat maintenance services represent an intermediate service which underpins several final ecosystem services including aquatic biomass production and recreation related services. In addition, chlorophyll concentrations, transparency and marine macroflora are correlated by feedback interactions. Chlorophyll concentration is a proxy for marine primary production. In turn, marine primary production plays an important role in ocean-atmosphere gas exchange processes, including carbon capture and oxygen production ((global) climate regulation). Regarding carbon storage and burial, the situation is mixed. While carbon burial increases as primary production increases, a reduction in carbon storage follows declines in ecosystem components such as seagrass beds and reefs that are often associated with advanced stages of eutrophication (Kermagoret et al., 2019; O'Higgins and Gilbert, 2014).
Furthermore, primary production is essential for the production and maintenance of the very biomass that supports marine food webs, and thus the ecosystem service here identified as ‘regulation and maintenance of marine food webs’ (O'Higgins and Gilbert, 2014). At more advanced stages of eutrophication, hypoxic conditions cause mass mortality of fish and invertebrates either directly, or indirectly through reduced prey abundance. This in turn results in a significant reduction in biomass supply (Crowe and Frid, 2015). The reduction of dissolved oxygen in bottom waters, while leading to a decline in the abundance of macrobenthic animals and an increase in the flow of energy through the microbial food web, leads to a reduction in the flow of energy through metazoan food webs that support mobile consumers belonging to higher trophic levels, a change that may reduce the ability to produce and supply biomass of interest to fisheries (Malone and Newton, 2020). In addition, changes in habitat conditions alter the composition of fish communities towards less valued species and cause the loss of nursery and feeding grounds for commercially important fish species (Crowe and Frid, 2015).
It should be mentioned that the water quality regulation service is also impacted following eutrophication. Some of the marine ecosystem components that contribute most to this service are the photosynthetic ones: phytoplankton, macrophytes, macroalgae and microphytobenthos. Of these, phytoplankton is the component that contributes most to primary productivity and hence nutrient uptake. However, in the presence of excess nutrients (eutrophication), this service can be compromised. Phytoplankton can grow in a detrimental way by impacting the surrounding marine environment, for example leading to hypoxia and/or anoxia in the benthic environment. As the presence of nutrients increases, the nutrient-limited phytoplankton grow exponentially. However, a threshold is reached where excessive increases in nutrient concentration begin to have a negative impact on the surrounding environment (Kermagoret et al., 2019). The service is considered to be negatively impacted when the negative effects of excessive nutrient input extend beyond the ability of ecological components (such as phytoplankton) to process them, and lead to other associated impacts (such as a decrease in dissolved oxygen) on other ecosystem components such as benthos (Culhane et al., 2020).
The study conducted by Kermagoret et al., (2019), in which impacts on bundles of ecosystem services along eutrophication gradients were observed, also showed that cultural ecosystem services are negatively affected, as events such as Ulva sp blooms affect recreational activities, spatial identity and emblematic biodiversity (thus also impacting the service here identified as spiritual, artistic, and symbolic services). Furthermore, it was observed how the degradation of marine ecosystem components such as seagrass beds and reefs can impact the provision of the ecosystem service of coastal protection (Kermagoret et al., 2019; Malone and Newton, 2020). Seagrass beds have the capacity to attenuate waves and slow currents, while reefs can dissipate a significant proportion of wave energy. Indeed, eutrophication is known to induce deterioration and gradual disappearance of elements such as seagrass beds and reefs (Kermagoret et al., 2019).
Culhane, F. E., Frida, C. L. J., Gelabert, E. R., Piet, G., White, L., and Robinson, L. A. (2020). Assessing the capacity of European regional seas to supply ecosystem services using marine status assessments. Ocean Coast. Manag. 190:105154.
Crowe, T.P., Frid, & C. L. J. (Eds.) (2015), Marine Ecosystems: Human Impacts on Biodiversity, Functioning and Services Cambridge University Press
EEA (2015) The European environment — state and outlook 2015: synthesis report, European Environment Agency, Copenhagen
Kermagoret, C., Claudet, J., Derolez, V., Nugues, M., Ouisse, V., Quillien, N., Baulaz, Y., Le Mao, P., Scemama, P., Vaschalde, D., Denis, B., and Mongruel, R. (2019). How does eutrophication impact bundles of ecosystem services in multiple coastal habitats using state-and-transition models. Ocean & Coastal Management. 174, pp. 144-153. https://doi.org/10.1016/j.ocecoaman.2019.03.028.
Limburg, K., Breitburg, D., Swaney, D., and Jacinto, G. (2020). Ocean Deoxygenation: A Primer. One Earth. 2. 24-29. https://doi.org/10.1016/j.oneear.2020.01.001.
Malone, T., and Newton, A. (2020). The Globalization of Coastal Eutrophication: Causes and Consequences. Frontiers in Marine Science, 7, 670, https://doi.org/10.3389/fmars.2020.00670
O'Higgins T.G., Gilbert A.J. (2014) Embedding ecosystem services into the Marine Strategy Framework Directive: Illustrated by eutrophication in the North Sea, Estuarine, Coastal and Shelf Science, Vol. 140,2014, pp. 146-152,ISSN 0272-7714, https://doi.org/10.1016/j.ecss.2013.10.005
State | Response |