Impacts on the provision of ecosystem services by pelagic habitats
Given the current changes occurring in pelagic habitats, in no case is a positive outcome observed or expected for the ecosystem services they provide. Climate change represents the most important pressure on pelagic habitats, but pressures related to nutrient inputs, eutrophication, fishing, introduction of non-indigenous species and other human activities can also impact pelagic habitats. The provision of marine ecosystem services is intrinsically linked to the dynamics of pelagic habitats. The holistic nature of the interactions between physical and biological systems that characterise pelagic habitats means that adverse changes in pelagic characteristics can affect the provision of ecosystem services to the entire marine ecosystem.
This section evaluates the impact that changes in the environmental state of pelagic habitats, as described in the State section, have on the ecosystem services that the North-East Atlantic provides. The section was developed through a literature review combined with expert judgement, using the same methodology across all Thematic Assessments. Several workshops involving ecosystem service experts and pelagic habitat experts were held to discuss and agree the presented results. A detailed description of the anthropogenic pressures that impact pelagic habitat state, and thus the provision of ecosystem services, are provided in the Pressure section. The following provides further details on the role that pelagic habitats (and their state) have in relation to the provision of ecosystem services outlined in Figure I.1.
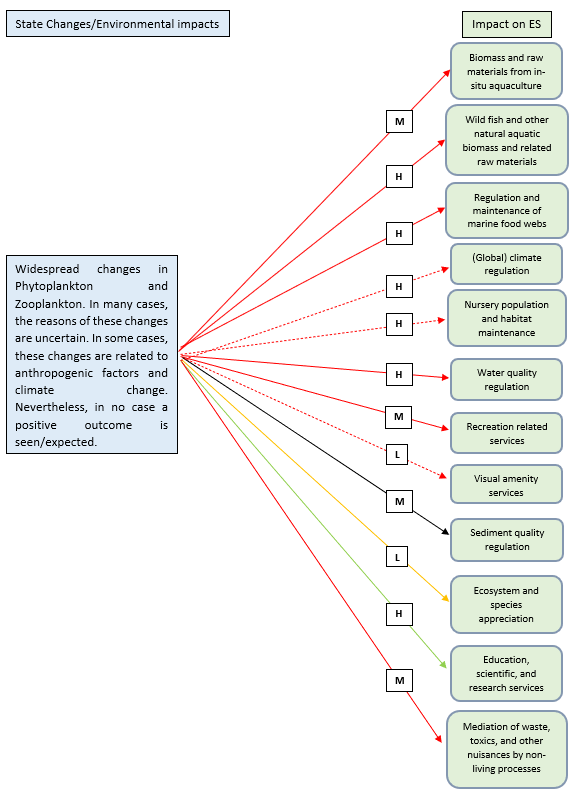
Figure I.1: Schematic depicting the 'State (changes)’ - ‘Impact on ES’ linkages for the Pelagic Habitats thematic assessment. The ecosystem services shown are those considered most relevant in relation to the Pelagic Habitats thematic assessment. Each arrow also denotes an expert judgement estimate of the nature and magnitude of the impact (black arrow = recognition of the existence of the impact but no clear negative or positive impact (uncertain nature); yellow arrow = neutral impact; red arrow = negative impact; red dashed arrow = potential negative impact; green arrow = positive impact; H = high impact; M = medium impact; L = low impact)
Detailed rationale for the role of pelagic habitats (and their state) in relation to the provision of ecosystem services
To assess pelagic habitat status for OSPAR QSR 2023, three indicators based on species (PH3 indicator ‘Changes in Plankton Diversity’), functional groups (PH1/FW5 indicator ‘Changes in Phytoplankton and Zooplankton Communities’), and community abundance/biomass (PH2 indicator ‘Changes in Phytoplankton Biomass and Zooplankton Abundance’) were identified. These indicators provide complementary information on the status and functioning of plankton communities, and for a solid overall assessment of pelagic habitat they should be considered simultaneously (OSPAR CEMP, 2018).
In the previous schematic, the message presented within the “State change” box was formulated by the Pelagic Habitats experts. This key message, obtained from an integrated consideration of the results obtained from the different indicators employed in QSR 2023 for the State assessment of Pelagic Habitats, concisely conveys the overall observed state (change) of the pelagic habitats in the OSPAR Maritime Area as a whole.
In the context of OSPAR IA 2017, the assessments demonstrated that for the most recent period (post-2000) zooplankton abundance tended to decrease while phytoplankton biomass tended to increase (OSPAR, 2017i). Additionally, OSPAR assessments of planktonic lifeforms (functional groups) showed that plankton communities (phytoplankton and zooplankton) had experienced significant changes, indicating possible alterations in pelagic habitats and marine ecosystem functioning (OSPAR, 2017i). However, although these assessments demonstrated that important changes had occurred, they were only preliminary assessments, and it is difficult to draw conclusions about direction, implications for the overall marine ecosystem dynamics, and consequently the provision of ecosystem services by pelagic habitats for the OSPAR Maritime Area (OSPAR, 2017i). In the context of the latest OSPAR QSR 2023 assessments, widespread changes in phytoplankton and zooplankton were observed. In some cases, the drivers of these changes are uncertain. In some cases, these changes are related to direct anthropogenic factors as well as climate change. Nevertheless, in no case is a positive outcome observed or expected.
By presenting and describing the ecosystem services underpinned by phytoplankton and zooplankton, and in general by pelagic habitats and their components, it is possible to develop informed assumptions as to which ecosystem services may be impacted (and how) because of major changes in phytoplankton and zooplankton abundance, community functioning, and the overall state of pelagic habitats which may result from environmental impacts related to human-induced pressures (Bedford et al., 2020). The following provides an elaboration on the contribution by plankton, and more generally by pelagic habitats, to the provision of the ecosystem services illustrated in the previous section. In the case of this thematic assessment, it should be noted that climate change represents the most important pressure on pelagic habitats, but pressures related to nutrient inputs, eutrophication, fishing, introduction of non-indigenous species and other human activities can also impact pelagic habitats (see: Trends in New Records of Non-indigenous Species Introduced by Human Activities and the other pressure-related thematic assessments for more details).
The dynamics of the interaction between physical and biological systems underpin the functioning of pelagic habitats. The physical system refers to the entire water column and its gradients of temperature, salinity, density, light, particles, and nutrients. The physical system significantly influences the distribution of pelagic biological communities. Pelagic habitats support biodiversity ranging in size from sub-millimetres (i.e., plankton) to metres (i.e., marine mammals) that involves primary production (e.g., phytoplankton), covers all trophic levels and regulates the physical and chemical conditions of the water (e.g., carbon sequestration) (Magliozzi et al., 2021). Key examples of interactions between pelagic physical and biological systems include the fluxes of matter and energy that sustain food webs, the interactions between different habitats (e.g., benthic-pelagic coupling), climate regulation through atmospheric oxygen production, and carbon sequestration. Many oceanic ecosystem services are mediated by plankton (both phytoplankton and zooplankton) and thus plankton are also used to track changes in upper trophic levels and top-down and bottom-up pressures. The holistic nature of the interactions between physical and biological systems that characterise pelagic habitats means that adverse changes in pelagic characteristics can affect the provision of ecosystem services to the entire marine ecosystem (Magliozzi et al., 2021). Indeed, as argued by Santora et al. (2021), the provision of marine ecosystem services is intrinsically linked to the dynamics of pelagic habitats. Consequently, given the multitude of interacting communities and the innumerable dynamics at play, the task of describing all ecosystem functioning and the resulting ecosystem services relating to the pelagic realm remains challenging (Dickey-Collas et al., 2017). Also, given the complexity of ecological dynamics within pelagic habitats and between habitats (e.g., benthic-pelagic coupling), all the ecosystem services considered in this work could potentially be considered as provided directly or indirectly by pelagic habitats, their ecological components and their interactions with the surrounding marine environment. Nevertheless, as stated by Dickey-Collas et al., (2017), key ecosystem services provided by pelagic habitats can be identified.
Life-cycle maintenance service, which in the most recent CICES v.5.1 classification (Haines-Young and Potschin, 2018) and in the ecosystem services considered in this report is represented by the regulation service nursery population and habitat maintenance (including the protection of natural gene pools), is considered one of the essential ecosystem services in relation to pelagic habitats. Indeed, pelagic habitats act as breeding (including spawning and mating), nursery and feeding grounds, as well as migration and advection routes, thus contributing to the maintenance of habitats and their biodiversity, both for species that live constantly in the water column and species that live in it only for specific stages of their life cycle (Dickey-Collas et al., 2017).
Pelagic habitats are crucial for nourishing the oceans through nutrient cycling, thus contributing directly and indirectly to supporting the growth of biomass in the various trophic levels that make up food webs (‘regulation and maintenance of marine food webs’). Also, one of the most tangible ecosystem services from pelagic habitats is represented by fish stocks (wild fish and other natural aquatic biomass and related raw materials) (Hays et al., 2005). In addition, through processes of ocean-atmosphere gas exchange regulation, the ocean is a net sink of atmospheric carbon dioxide ((global) climate regulation). Ocean warming due to climate change pressures is expected to reduce the provision of this ecosystem service by pelagic habitats (Dickey-Collas et al., 2017).
Other ecosystem services that are underpinned by the physical and biological processes taking place in the water column are water quality regulation and the mediation of waste, toxins and other nuisances by abiotic processes. Under natural conditions, organic waste entering the water undergoes degradation processes that include remineralisation by pelagic communities of microbes and accumulation in biomass. As for more persistent contaminants, their toxicity can be reduced through abiotic processes occurring at the ocean-atmosphere interface, such as photochemical and hydrolytic degeneration reactions. Phytoplankton, zooplankton and fish also contribute, albeit to a lesser extent, to further waste degradation in the water column. In addition, abiotic processes such as dispersion and advection help to provide export pathways for wastes that thus avoid being concentrated in hot spots (Watson et al., 2016). The provision of these services, in similar fashion to the other mentioned ecosystem services, may be reduced and/or compromised as a result of major alterations in physical-biological dynamics following environmental impacts related to pressures such as eutrophication, climate change and excessive input of pollutants etc (see pressure-related thematic assessments for more details).
The pelagic realm provides all cultural ecosystem services ranging from the scientific knowledge that can be obtained from the different pelagic environments, educational value, exploration and related technological developments, to services including literature, entertainment, ethical considerations, tourism and spiritual health and wellbeing (Thurber et al., 2014).
Half of the primary production that supports food webs is provided by photosynthetic processes carried out by microbial and phytoplankton communities in the water column. Changes in these communities can in turn affect the survival of organisms at higher levels of the food web. This is one of the main reasons why the ecological processes provided by pelagic habitats contribute directly to the provision of various ecosystem services and indirectly support the provision of ecosystem services also provided by organisms at other levels of the food web (EEA, 2015).
Marine phytoplankton are a well-known provider of ecosystem services, crucially contributing to the climate cycle and functions of the marine ecosystem and beyond. Phytoplankton are one of the main marine ecosystem components that contribute most to providing the ecosystem service of (global) climate regulation. Some phytoplankton species produce dimethylsulphoniopropionate (DMSP), a precursor to dimethyl sulphide (DMS), important for the process of cloud formation. Phytoplankton are also a major contributor to the carbon fixation process and its sequestration in sediments and ocean depths, capturing carbon dioxide from the atmosphere (Tweddle et al., 2018). At the same time, as a food source for pelagic herbivores (including larval stages), phytoplankton also form the basis of marine food webs (‘regulation and maintenance of marine food webs’), supporting the production and supply of biomass from the upper trophic levels, including biomass produced in marine aquaculture or mariculture contexts (wild fish and other natural aquatic biomass and related raw materials and biomass and raw materials from in situ aquaculture). Spatial variability in primary productivity and phytoplankton abundance can influence the distributions of upper trophic levels, including fish, birds and marine mammals and, indirectly, the local provision of ecosystem services by these organisms (see: Marine Birds Thematic Assessment , Marine Mammals Thematic Assessment , Fish Thematic Assessment for more details about the range of ecosystem services provided by marine birds, marine mammals, and fish; Tweddle et al., 2018).
It should also be noted that shelf areas support most oceanic primary production but are, at the same time, the areas most directly affected by human activities and related pressures (e.g., offshore oil and gas, wind energy, wave and tidal renewable energy, aquaculture, aggregate dredging and waste dumping; see these sections in the pressure-related OSPAR thematic assessments and Tweddle et al., 2018). The role played by anthropogenic pressures and their impacts in relation to food webs and components such as phytoplankton must be kept in mind. Given the importance of pelagic environments in supporting the entire marine ecosystem, cumulative impacts resulting from human-induced pressures (addressed in the pressure-related thematic assessments) can alter the physical and biological dynamics governing the water column and in turn have a negative cascading effect on the provision of ecosystem services by other habitats that depend on them. Phytoplankton are essential in benthic-pelagic coupling. Benthic-pelagic coupling is crucial for the functioning and balance of food webs as it involves functions from nutrient cycling to energy transfer between habitats (Griffiths et al., 2017). A climate change-related increase in water temperature may cause changes in the timing and decrease in the magnitude of phytoplankton blooms, which in turn may lead to a decrease in the transport of organic matter to the benthos and reduce the release of inorganic nutrients from the marine sediment. Changes in coupling between pelagic and benthic systems can in turn lead to a decrease in benthic productivity and reproduction.
Regarding OSPAR pelagic habitat indicators ( Changes in Phytoplankton and Zooplankton Communities , Changes in Phytoplankton Biomass and Zooplankton Abundance ), changes in the balance of 'planktonic lifeforms' (phytoplankton and zooplankton), namely the changes in functional group abundance, are analysed to assess the state of the lower trophic levels in the North-East Atlantic. This can be used to detect changes in community functioning, which in turn can inform changes in ecosystem services (Bedford et al., 2020; McQuatters-Gollop et al., 2019). In addition, zooplankton are directly linked to the higher trophic levels representing primary consumers. The presence and abundance of zooplankton, and their predator-prey relationship with other trophic levels, influence fish recruitment and the production of wild biomass in higher trophic levels (wild fish and other natural aquatic biomass and related raw materials) and the biomass produced in mariculture contexts (biomass and raw materials from in situ aquaculture). Zooplankton’s contribution to sustaining the balance of food webs (‘regulation and maintenance of marine food webs’) is also detectable in their role as a grazer for phytoplankton and bacteria and as a provider of nitrogen and phosphorus (nutrient cycling), which has positive effects on the primary productivity of phytoplankton. In addition, zooplankton, through processes such as the export of particles by grazing, the fractioning of sinking particles, and the transport of particulate organic carbon at depth through their diel vertical migration, play a crucial role in the functioning of the oceanic biological carbon pump that contributes to regulating atmospheric carbon dioxide levels ((Global) climate regulation) (Lomartire et al., 2021). Regarding plankton diversity, species composition and abundance are influenced by changes in physical and chemical environmental conditions. Human-induced disturbances can lead to changes in plankton diversity because only some species are able to tolerate altered habitat conditions. Consequently, plankton diversity, as well as plankton lifeform biomass and abundance, will differ between disturbed and undisturbed communities (OSPAR, 2017i).
Examples of the impacts that may result from human-induced pressure are the negative changes in plankton communities due to local-scale inputs of nutrients (e.g., from farming run-off, sewage, dredging). Changes in plankton lifeforms can result from inputs and increases in nutrients. The onset of eutrophication leads to an increase in phytoplankton biomass. However, the composition of the phytoplankton community becomes more uneven, with the disappearance of some species and the predominance of opportunistic species. This leads to a decrease in species diversity due to competitive exclusion. However, this depends on the level of eutrophication: whereas, with a slight increase in eutrophication, the competition loosens, resulting in greater diversity, when there is a further increase in eutrophication, the diversity decreases again due to stress (McQuatters-Gollop et al., 2009; Spatharis et al., 2007). Either way, eutrophication, by impacting plankton and primary productivity, can lead to less desirable food web conditions. Localised changes in nutrients and plankton distribution can also result from changes to hydrological conditions following coastal development and the introduction of infrastructures.
Changes in the balance of food webs can occur because of injury, mortality and/or extraction associated with overfishing (commercial and recreational). The removal of predatory fish can lead to forage fish (plankton consumers) becoming too abundant. In turn, zooplankton are over-consumed, leading to imbalances in plankton communities (changes in plankton biomass and/or abundance). This may then result in changes (reductions) in the energy available for supporting higher trophic levels.
Bedford, J., Ostle, C., Johns, D. G., Atkinson, A., Best, M., Bresnan, E. and McQuatters‐Gollop, A. (2020). Lifeform indicators reveal large‐scale shifts in plankton across the North‐West European shelf. Global Change Biology, 26(6), 3482-3497.
Dickey‐Collas, M., Link, J. S., Snelgrove, P., Roberts, J. M., Anderson, M. R., Kenchington, E. and Johannesen, E. (2022). Exploring ecosystem‐based management in the North Atlantic. Journal of Fish Biology, 101(2), 342-350.
EEA (2015). State of Europe's seas. EEA Report No 2/2015, European Environment Agency. Retrieved September 23, 2021, from http://www.eea.europa.eu/publications/state-of-europes-seas
Griffiths, J. R., Kadin, M., Nascimento, F. J., Tamelander, T., Törnroos, A., Bonaglia, S. and Winder, M. (2017). The importance of benthic–pelagic coupling for marine ecosystem functioning in a changing world. Global change biology, 23(6), 2179-2196.
Haines-Young, R. and Potschin ,M.B.(2018). Common International Classification of Ecosystem Services (CICES) V5.1 and Guidance on the Application of the Revised Structure. Retrieved from https://cices.eu/content/uploads/sites/8/2018/01/Guidance-V51-01012018.pdf
Hays, G. C., Richardson, A. J. and Robinson, C. (2005). Climate change and marine plankton. Trends in ecology & evolution, 20(6), 337-344.
Lomartire, S., Marques, J. C. and Gonçalves, A. M. M. (2021). The key role of zooplankton in ecosystem services: A perspective of interaction between zooplankton and fish recruitment. Ecological Indicators, 129, 107867. doi: https://doi.org/10.1016/j.ecolind.2021.107867
Magliozzi, C., Druon, J. N., Palialexis, A., Aguzzi, L., Alexande, B., Antoniadis, K. and Zervoudaki, S. (2021). Pelagic habitats under the MSFD D1: scientific advice of policy relevance. European Commission, Luxembourg.
McQuatters-Gollop, A., Atkinson, A., Aubert, A., Bedford, J., Best, M., Bresnan, E. and Tett, P. (2019). Plankton lifeforms as a biodiversity indicator for regional-scale assessment of pelagic habitats for policy. Ecological Indicators, 101, 913-925.
McQuatters-Gollop, A., Gilbert, A. J., Mee, L. D., Vermaat, J. E., Artioli, Y., Humborg, C. and Wulff, F. (2009). How well do ecosystem indicators communicate the effects of anthropogenic eutrophication? Estuarine, Coastal and Shelf Science, 82(4), 583-596. doi: https://doi.org/10.1016/j.ecss.2009.02.017
OSPAR (2017i). OSPAR Intermediate Assessment 2017: Habitats. Retrieved from: https://oap.ospar.org/en/ospar-assessments/intermediate-assessment-2017/biodiversity-status/habitats/
OSPAR CEMP (2018). OSPAR CEMP Guideline. Common indicator: PH1/FW5 Plankton lifeforms (OSPAR Agreement 2018-07). Retrieved from: https://www.ospar.org/documents?v=39001
Santora, J. A., Schroeder, I. D., Bograd, S. J., Chavez, F. P., Cimino, M. A., Fiechter, J. and Field, J. C. (2021). PELAGIC BIODIVERSITY, ECOSYSTEM FUNCTION, AND SERVICES. Oceanography, 34(2), 16-37.
Spatharis, S., Danielidis, D. B. and Tsirtsis, G. (2007). Recurrent Pseudo-nitzschia calliantha (Bacillariophyceae) and Alexandrium insuetum (Dinophyceae) winter blooms induced by agricultural runoff. Harmful Algae, 6(6), 811-822.
Thurber, A. R., Sweetman, A. K., Narayanaswamy, B. E., Jones, D. O., Ingels, J. and Hansman, R. L. (2014). Ecosystem function and services provided by the deep sea. Biogeosciences, 11(14), 3941-3963.
Tweddle, J. F., Gubbins, M. and Scott, B. E. (2018). Should phytoplankton be a key consideration for marine management? Marine Policy, 97, 1-9.
Watson, S. C. L., Paterson, D. M., Queirós, A. M., Rees, A. P., Stephens, N., Widdicombe, S. and Beaumont, N. J. (2016). A conceptual framework for assessing the ecosystem service of waste remediation: In the marine environment. Ecosystem Services, 20, 69-81. doi: https://doi.org/10.1016/j.ecoser.2016.06.011
State | Response |