Responding to the climate emergency: mitigation, resilience, and adaptation
The OSPAR Contracting Parties will need to take action to reduce global warming in order to comply with the UNFCCC Paris Agreement (i.e. to limit the rise of global average temperatures to well below 2°C, and ideally below 1.5°C above pre-industrial levels), to adapt to climate change impacts (those that are already emergent and those that are locked in because of greenhouse gas emissions to date and anticipated in future years) and to increase the resilience of human and biological systems. To date, no specific OSPAR measures to mitigate or adapt to climate change or to increase resilience are in place, although the NEAES 2030 has outlined an ambitious strategy by the OSPAR Contracting Parties intended to make significant progress on climate change and ocean acidification. Areas of opportunity for mitigation include offshore renewable energy, carbon capture and storage and protection and restoration of natural carbon stores. Through effective management of marine space and of human pressures on the marine ecosystem, the OSPAR Contracting Parties may be able to support adaptation to climate change and enhance the resilience of the marine ecosystem.
Climate change mitigation, adaptation and resilience in the OSPAR context
The ocean plays a pivotal role in climate regulation through its uptake of CO2 and heat from the atmosphere. The ocean currents transport considerable energy around the globe. Ocean currents work like conveyor belts that transport vast quantities of heat around our planet, maintaining many of the Earth’s climate zones. The uptake of heat by the oceans has significantly slowed down climate change and mitigated global warming. Through the processes of the marine carbon pump, atmospheric CO2 is stored in plants, animals and marine sediments in the form of organic carbon. Absorbing more than a quarter of global anthropogenic CO2 emissions, the ocean is one of the biggest natural carbon sinks on our planet.
However, the ocean is also vulnerable to the impacts of anthropogenic pressures and the effects of climate change. Mitigation (the reduction of greenhouse gas emissions or the enhancement of their sinks) and adaptation (the adjustment to actual or anticipated climate) are required in order to maintain and build the resilience of the ocean and its ecosystems against these threats. Resilience is the capacity of a system to absorb disturbance without losing its essential function. Mitigation and adaptation are processes which can either maintain or build resilience. OSPAR still needs to adopt further specific measures to mitigate or adapt to climate change or to increase resilience. The NEAES 2030 has outlined OSPAR’s ambitions to support climate action across its Contracting Parties. The Ocean Acidification Other Assessment has highlighted a number of mitigation and adaptation responses that are effective for ocean acidification. Below, a broader overview is provided.
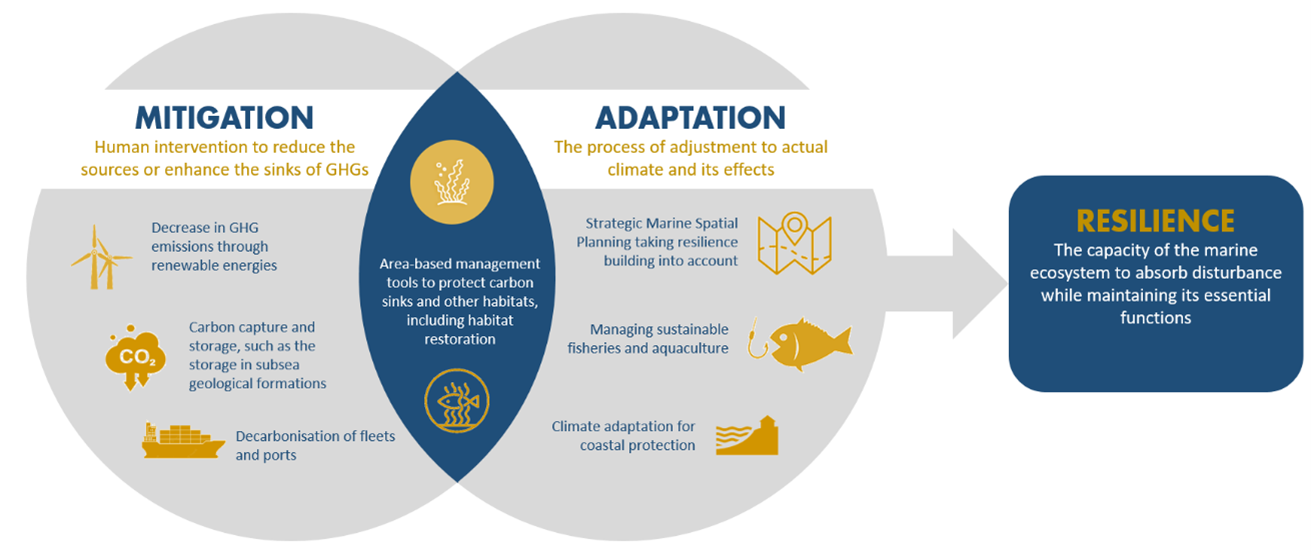
Climate change mitigation
Mitigation is defined as “A human intervention to reduce emissions or enhance the sinks of greenhouse gases” (IPCC, 2022). Each year, the global ocean absorbs approximately one quarter (Friedlingstein et al., 2021) or possibly more (Watson et al., 2020) of the carbon dioxide released to the atmosphere from human activities (Friedlinstein et al., 2021). On a global scale, 12% of the mitigation required by 2030 to ensure that warming does not surpass 1.5˚C could be provided by ocean-based initiatives (Hoegh-Guldberg et al., 2019). The growing role of all actors in the global effort to address climate change was recognised in the recent IPCC report on Mitigation of Climate Change (IPCC, 2022). This clearly also applies to marine initiatives, and can encompass national, regional and global climate policies that are boosted by the mitigation actions of citizens, businesses, cities, and local initiatives. Regional level initiatives that can reduce carbon emissions or increase carbon sinks are considered below. The same IPCC report also discusses wider policy considerations and their potential outcomes and implications.
Changing energy strategies (wind)
Electricity and heat account for 23% of total global greenhouse gas emissions (IPCC, 2022), and many countries are changing their energy strategies to reduce these. Renewable energy generation in the marine environment has grown substantially, mainly through the development of offshore wind farms, although wave and tidal energy are also being considered by some nations where conditions are suitable (high wave exposure, large tidal range or strong tidal streams).
Within the OSPAR Maritime Area, growth in offshore wind energy generation has been substantial, and a further significant increase is expected in the next decade, especially in Regions II and III.
The substantial current and anticipated growth of the offshore renewable energy sector also requires suitable consideration of the ecological impacts so as to ensure sustainable development. OSPAR will progress this under Strategic Objective S12.O4: “By 2023 OSPAR will develop common principles and by 2024 develop guidance to promote and facilitate sustainable development and scaling up of offshore renewable energy in such a way that cumulative environmental impacts are minimised”. Knowledge gaps remain, particularly around cumulative effects, although a number of major evidence programmes are in progress to address this aspect. OSPAR’s Intersessional Correspondence Group on Offshore Renewable Energy Development (ICG-ORED) was convened for the first time in 2022 and aims to contribute to this objective by producing a regional sea approach to the assessment of the cumulative effects associated with the development of offshore renewable energy. This approach could then guide future measures and actions across the OSPAR Contracting Parties.
Carbon capture and storage (CCS)
Carbon capture and storage (CCS) (see: glossary) is the capture of carbon dioxide from anthropogenic source emissions and its subsequent transport to and storage in underground geological storage sites. This is a human activity intended to reduce atmospheric carbon dioxide emissions. In 2007, the OSPAR Commission endorsed CCS in subsea geological formations, provided that carbon dioxide streams stored in geological formations are retained in those formations permanently (OSPAR Decision 2007/02). Under this Decision, storage of carbon dioxide should be authorised only if it does not lead to negative consequences for the marine environment, human health and other uses of the maritime area.
The storage of CO2 in geological formations, including depleted oil and gas reservoirs and saline aquifers, is an emerging offshore activity. There are two large-scale CO2 storage projects currently operating in the OSPAR Maritime Area (see: Offshore Industry Thematic Assessment ). There is the risk of CO2 leakage from a storage site, and should it occur it may have negative effects on the receptors in the marine environment. Other negative effects may include an increase in underwater noise from activities to monitor the integrity of sub-seabed storage (see: Underwater Noise Thematic Assessment ).
Work by the IPCC has recognised that pathways to limit global warming to 2°C or below may require the deployment of a certain amount of carbon dioxide removal technology, such as CCS, particularly in the short-term. However, it is widely recognised that these should not be considered the sole method for reducing greenhouse gas emissions. For OSPAR Contracting Parties that are also Parties to the London Protocol (1996 Protocol to the Convention on the Prevention of Marine Pollution by Dumping of Wastes and Other Matter, 1972), Resolution LP.5(14) recognises that CCS should be considered part of a portfolio of options and should not be considered as a substitute for other measures to reduce carbon dioxide emissions. The Decision also stresses that the disposal of carbon dioxide streams into sub-seabed geological formations does not remove the obligation to reduce the need for such disposal or the commitments undertaken in the framework of the UNFCCC to reduce greenhouse gas emissions.
As part of NEAES 2030, under Strategic Objective S12.O3, OSPAR will review and assess the adequacy of monitoring techniques and the effectiveness of measures to ensure that carbon dioxide streams are retained permanently in the storage complex and will not lead to any significant adverse consequences (as above).
De-carbonisation of fleets
Maritime transport plays a key role in the facilitation of international trade, accounting for almost 80% of worldwide goods transportation (European Commission, 2021). While shipping is the most carbon-efficient mode of transport per tonne/kilometre, it produced 2.9% of annual global greenhouse gas emissions in 2018, representing 1 076 million tonnes of CO2. Approximately 85% of the total CO2 emissions from international shipping comes from ships of 5,000 gross tonnage or above (IMO, 2018; IMO 2020). High densities of shipping occur in the OSPAR Maritime Area, with the highest occurring in the English Channel, southern and eastern North Sea and the entrance to the Mediterranean (see: Shipping and Ports Feeder Report )
Additionally, ships produce black carbon particles when burning heavy fuel oil, which are emitted into the environment via their exhaust fumes. These particles contribute to the warming of the Earth’s atmosphere. When they fall on snow, glacier ice or sea ice, their warming impact is 7 to 10 times greater than on land, as their reflectivity (albedo) is reduced and consequently their absorption of heat increases. According to the International Council on Clean Transportation, approximately 7 to21% of global shipping’s climate warming impacts can be attributed to black carbon over a 20-year Global Warming Potential time frame – the remainder being mostly CO2, followed by methane (CH4). Black carbon emissions from shipping increased by 85% between 2015 and 2019.
The IMO is responsible for the global regulation of international shipping and has a key role in ensuring that lives at sea are not put at risk, including by ensuring security of shipping, and that the environment is not polluted by ships’ operations. Since 2011 (IMO, 2011), the IMO has developed mandatory regulations to address greenhouse gas emissions from ships through a number of resolutions focusing on mandatory technical and operational measures to improve energy efficiency. A revised strategy with strengthened objectives is to be adopted by the IMO in July 2023, while the initial IMO Strategy on reduction of greenhouse gas emissions from ships agreed in 2018 has the overall objective of full decarbonisation and the ambition to reduce the emissions from international shipping by at least 50% by 2050, compared with 2008 (IMO, 2018).
To date, the maritime sector is not on track to achieve the emissions reductions needed to achieve its commitments under the Paris Agreement. In the context of the IMO strategy, new measures are being developed and their prospects considered. Potential measures include support for the uptake of alternative low-carbon and zero-carbon fuels, in the form of guidelines on the lifecycle greenhouse gas intensity of marine fuels, low-carbon fuel standards and carbon pricing, as well as further energy efficiency measures such as fleet improvement and efficient new ship design, speed optimization and reduction, addressing emissions of methane and Volatile Organic Compounds, and others.
In the European context, the 2020 EU Communication on a Sustainable and Smart Mobility Strategy aims to achieve zero pollution of air and water from shipping in emission control areas and to bring the first zero-emission ocean-going vessels to market by 2030 (European Commission, 2020). Under the European Green Deal, a series of legislative proposals have been formulated with a view to reducing net greenhouse emissions, several of which address the maritime transport sector, such as the inclusion of shipping in the EU Emissions Trading System (ETS), the FuelEU Maritime (a carbon fuel standard for marine fuels), the Alternative Fuels Infrastructure Directive, the revision of the renewable energy directive (RED) and the revision of the Energy Tax Directive.
Ports
Ports are crucial to providing the infrastructure for maritime transport and play an essential role in the development of many maritime sectors. In 2018, the gross weight of goods handled in OSPAR ports was around 2,4 billion tonnes, with the number of containers involved slightly higher than 10 years previously (see: Shipping and Ports Feeder Report ).
The future of ports lies in their key role as ‘…energy hubs (for integrated electricity, hydrogen and other renewable and low-carbon fuels systems), for the circular economy (for collecting, transhipping and disposing of waste from ships and other port industries, and for decommissioning ships), for communication (for submarine cables), and for industry (as industrial clusters)’ (European Commission, 2021 ).
The greening of maritime transport and ports can contribute to the efforts to mitigate carbon emissions. The creation of zero-emission ports, for example, is one of the flagship initiatives of the European Commission’s sustainable and smart mobility strategy, promoting measures to encourage the deployment of renewable and low-carbon fuels and onshore power supply together with renewable energy and the greening of port services and operations. Another element helping decarbonisation is the use of smart digital solutions and autonomous systems, as they can optimise traffic flows and cargo handling in and around ports.
The gross tonnage of ships entering OSPAR ports has increased in the last ten years. The trend towards larger ships, while resulting in lower average transport costs, also necessitates ongoing dredging, new port infrastructure and the possible relocation of ports, and increases the potential for greater environmental impacts if a serious accident were to occur (see: Shipping and Ports Feeder Report ).
Blue carbon and marine sedimentary carbon stores
Some coastal and marine habitats can take up and store large quantities of carbon derived from atmospheric carbon dioxide through natural processes. Coastal blue carbon habitats include mangroves, salt marshes and seagrasses, which have high carbon burial rates on a per unit area basis and accumulate carbon in their soils and sediments (IPCC 2022, AR6). These ecosystems are recognised in the official international methodologies for inclusion in national greenhouse gas inventories. Seagrass meadows, mangrove forests and salt marshes are, per unit area, more effective stores of carbon than tropical forests. Despite their much smaller overall area, total global sequestration by these habitats is comparable to total global annual organic carbon storage by terrestrial forests (Mcleod et al., 2011).
Other habitats that have not yet been incorporated in the international standard methodology owing to lack of knowledge include coastal and non-coastal processes and ecosystems such as macro-algae (kelp) and carbon-rich coastal and shelf sea sediments. There is a need to improve the evidence on these habitats so as to make future greenhouse gas reporting and accounting more robust.
Marine sediments are important areas of natural carbon sequestration and storage and constitute the largest permanent sink for carbon on our planet (Berner and Berner, 2012). In the North Sea area, carbon stocks in muddy sediments (“mud carbon”) are two orders of magnitude higher than those in blue carbon ecosystems (Legge et al., 2020). Activities that result in disturbances to the seabed, such as dredging or trawling, can cause carbon dioxide to be released from sediments, but the processes are complex and the overall impact of carbon dioxide release and its destiny (sediment, water or atmosphere) remains uncertain. Several scientific projects in OSPAR Contracting Parties continue to build the evidence for potential blue and mud carbon habitats, including marine sediments, and threats from human activities and climate change.
Within NEAES 2030, the role of natural carbon storage in marine habitats, such as blue carbon, in helping mitigate climate change and resilience is recognised under the following strategic objectives:
- Strategic Objective 6: Restore degraded benthic habitats in the North-East Atlantic when practicable to safeguard their ecosystem function and resilience to climate change and ocean acidification.
- Strategic Objective S12.O1: By 2025 OSPAR will develop a regional approach to applying nature-based solutions for carbon storage and implement specific measures to protect and restore relevant carbon sequestration and storage habitats, such as seagrass beds, kelp forests and saltmarshes.
- Strategic Objective S12.O2: By 2025 OSPAR will take nature-based carbon storage into account when reviewing the criteria for the designation of marine protected areas, and reviewing the OSPAR List of threatened and / or declining species and habitats.
Many of these carbon-storing habitats provide additional benefits (beyond carbon sequestration or storage), including their roles as a habitat for species of conservation and commercial interest and in coastal flood protection. They thus also provide climate change adaptation responses in terms of income and subsistence from harvesting, and coastal infrastructure.
Adaptation
Adaptation is defined as “In human systems, the process of adjustment to actual or expected climate and its effects, in order to moderate harm or exploit beneficial opportunities. In natural systems, the process of adjustment to actual climate and its effects; human intervention may facilitate adjustment to expected climate and its effects.” (IPCC 2022, AR6). It requires persistent evaluation of climate-related risks and the implementation of proactive policies and measures, over time, to build resilience into our ecosystems and societies against the impacts of climate change. Adaptive actions should be risk-based, underpinned by an understanding of projected climate change, and informed by the existing vulnerabilities in our ecosystems and the human activities that take place in the marine environment.
Climate-smart marine management
Climate change is modifying the oceanographic conditions that underpin the structure and functioning of marine ecosystems. These evolving conditions will alter the spatial and temporal range of species and habitats and, in turn, the provision and distribution of vital goods and services they underpin. In combination with this, projected wind extremes, storm surges and increased wave energy may affect the distribution and sustainability of human activities such as fishing and aquaculture, maritime traffic, tourism, coastal infrastructure and maritime structures relating to offshore wind energy. The magnitude of the impacts will be regionally specific and will vary across different maritime sectors, requiring varying response and management options.
Adaptive management policies which integrate climate change into marine spatial planning (“Climate Smart MSP”) could enable maritime sectors, and marine protection efforts, to respond to future scenarios (Frazao-Santos et al., 2020). This could reduce the vulnerability of marine systems to changing ocean conditions by ensuring that the cumulative impacts of climate change and anthropogenic maritime uses such as development, pollution and resource extraction, are taken into consideration (Santos et al., 2020). To inform this process, it would be essential to improve knowledge and data on the possible impacts of climate change. This could include the identification of vulnerable species and ecosystems and possible future shifts in their geographical and temporal distribution. The identification of climate change refugia (areas with natural resilience, where the ecosystem remains within the boundaries of its present state), hotspots (where climate drives the ecosystem towards a new state, inconsistent with present usage), and bright spots (areas where oceanographic processes drive range expansion opportunities that may support sustainable growth in the medium-term) would also help support adaptation (Queirós et al., 2021).
The goals of the Paris Agreement include limiting the rise of global average temperatures to well below 2 ˚C, and ideally below 1,5 ˚C above pre-industrial levels. The expansion of renewable energy is one of the critical ways in which countries can help meet these obligations, the EU’s energy and climate targets and the ambitions of the EU Green Deal. Considering the level of growth in this sector, it may be important to balance the distribution of renewable energy installations in accordance with the likely climate-related changes in ecosystem functioning. Moreover, there may be need to adapt maritime structures to changes in wave frequency and intensity, during construction and then during ongoing management periods. Balancing renewable energy needs, the possible impacts of climate change and the cumulative impacts of anthropogenic pressures on the marine environment will require foresight in all marine spatial planning scenarios.
Food Security and sustainability
Fisheries and aquaculture provide food and nutrition globally while also contributing to the income and livelihoods of millions of people (FAO, 2018). Observed climate change is already affecting global food security through increasing temperatures, changing precipitation patterns and greater frequency of extreme events (Mbow et al., 2019). Impacts on marine systems, species redistribution and productivity are projected to challenge the provision of marine food by the mid-twenty-first century (IPCC, 2014).
Engaging in adaptation strategies that address climate change effects while concurrently considering other anthropogenic pressures will be essential to ensure the security and long-term sustainability of fisheries and aquaculture. Such strategies may include diversification within and across sectors, effective governance, improved management and conservation, and the continued development of low-input and low-impact aquaculture (FAO, 2018). Successful adaptation may depend strongly on the adaptive capacity of the industry, consideration of the correct options for localised pressures and the underlying environment, policy support and stakeholder involvement in the decision-making process.
Fisheries
The availability of fish stocks is strongly influenced by past and present fishing activities. Regional variations in the impacts of temperature and net primary production (an indication of the production at the base of the food web and ecosystem) have indicated that these two factors may also affect range shifts, growth, reproduction, the survival of fish stocks and species composition (Bahri, 2021; Bindoff et al., 2019; State section ).
Pursuing sustainable fishery practices and eliminating overfishing could reduce the risk caused by climate change and support the adaptation of fishery governance responses (Bindoff et al., 2019). Management frameworks that allow decisions to be tested, evaluated, reviewed and adjusted, on the basis of the observations of real time changes in climatic, environmental and fish stock conditions, could also greatly assist adaptation (Bahri, 2021).
To support such robust fisheries management, reliable knowledge provision will be essential (Bindoff et al., 2019). This includes data, modelling and projections of species shifts under forecasted climate scenarios. Such shifts in the range and distribution of fish stocks may require strong transboundary fisheries management together with adaptive allocation arrangements. Improved fishing gear and technology could also be considered in fisheries’ adaptation strategies.
Aquaculture
The impacts of climate change on aquaculture can include short and long-term losses of production or infrastructure due to extreme weather events, increased risk of disease, toxic algae and parasites, decreased productivity due to suboptimal conditions, limited access to feeds, reduced feed production (e.g. soy), scarcity of wild seed and increased perturbations such as eutrophication (Barange et al., 2018). Ocean acidification may also affect aquaculture, in particular shellfish, in the North-East Atlantic, and threaten larval development, wild seed recruitment, the bio-availability of contaminants and the resilience of adult populations.
As with fisheries management, strategies that are adaptive and include considerations of the negative effects of climate change while also considering the possible positive gains could limit overall impacts (Maulu et al., 2021). The adaptation options for aquaculture can include livelihood diversification, a shift to less vulnerable or more resilient species / techniques / areas, utilisation of local knowledge, and the introduction of insurance schemes among small-scale producers (see review in Maulu et al., 2021).
Climate adaptation for coastal protection
Sea-level rise and changes in prevailing weather (storminess, wave climate) will increase the occurrence of coastal flooding events and coastal erosion. Future projections show that compound flooding (a co-occurrence between storm surge and heavy precipitation and run-off) will also increase around the coasts of the OSPAR Maritime Area, with the largest increases along the North Sea coast (Bevacqua et al., 2019).
Coastal erosion, in particular, is a highly local and often episodic process, owing to the interplay between local coastal morphology, prevailing ocean conditions and meteorology. Adaptive actions for coastal protection will therefore require a site-specific approach taking into account local or sub-regional predictions of coastline response.
The range of measures for controlling flooding events includes hard engineering interventions (e.g. dikes, sea walls) and nature-based solutions (e.g. coastal vegetated ecosystems, biogenic reefs). Increasingly, preference is being given to “work with nature”, owing to the many associated co-benefits, whether environmental, social or economic. Examples of nature-based solutions in the coastal environment include the managed realignment of salt marsh, the restoration of oyster reefs, and protection and maintenance of natural dune systems. The implementation of nature-based solutions also calls for consideration of biosecurity in order to avoid the introduction of non-native invasive species.
Inland flood risk is also likely to increase due to climate change, and such events may cause acute pollution downstream in connected coastal and marine ecosystems. They could also have implications for nutrient loading (land run-off from agriculture and waste water treatment capacity), contaminants and litter (see: State section ).
The adaptation-maladaptation continuum – a cautionary note
In “Climate Change 2022: Impacts, Adaptation and Vulnerability”, the IPCC documents increased evidence of maladaptation across many sectors and regions since the previous assessment cycle (IPCC, 2022). Maladaptive actions have been defined by the IPCC as “actions that may lead to an increased risk of adverse climate-related outcome, including via increased greenhouse gas emissions, increased or shifted vulnerability to climate change, more inequitable outcomes, or diminished welfare, now or in the future.” In its latest report, the IPCC recognises that successful adaptation is not a “black and white” outcome, but instead involves a continuum of risk management in which successful adaptation and maladaptation can be considered the two extremes (New et al., 2022). Actions can score high or low against a range of outcomes, such as number of beneficiaries, ecosystem service benefits, equity outcomes, transformation and greenhouse gas emissions reduction. Examples of maladaptive actions in the context of OSPAR include lock-in caused by hard-engineered coastal defences or increased fossil fuel use in fisheries due to distributional shifts in target species. An adequate knowledge base, awareness of the long-term impacts and planning, and cross-sectoral and inclusive governance can help reduce maladaptation.
Resilience
Resilience is defined as “The capacity of interconnected [...] systems to cope with a hazardous event, trend or disturbance, responding or reorganising in ways that maintain their essential function [...]” (IPCC, 2022). A resilient ocean is thus one that is healthy and stable enough to withstand disturbances without losing its ecosystem functions. Such events could be due to climate change or other anthropogenic pressures that are threatening and changing marine ecosystems. OSPAR will support efforts to maintain and increase the resilience of marine ecosystems through a range of instruments. The following section discusses some of these measures.
Managing non-climate anthropogenic pressures to increase resilience
The combined effects of multiple pressures, including those from human activities, reduce the resilience of the marine ecosystem, habitats and species, including their ability to withstand and adapt to the impacts of climate change. It follows that the management of non-climatic pressures is also a critical tool for OSPAR to use in supporting climate change resilience. Examples of such management are control of nutrient inputs, addressing eutrophication effects, reducing the release of contaminants and radioactive substances, reducing marine and other litter and implementing an ecosystem-based management approach.
Area-based management tools as instruments for building resilience to climate change
Area-based management tools (ABMTs) enable the application of management measures to a specific area to achieve a desired policy outcome. ABMTs can focus on specific activities (e.g. fishery closures, pollution management) or on the coordination and management of several activities within an area (e.g. integrated coastal zone management, marine spatial planning). A wide variety of area-based management tools exists, each with their own purpose, mandate and authority (UN Environment, 2018). These include:
- Sectoral ABMTs, including the IMO’s Particularly Sensitive Sea Areas (PSSAs), traffic routeing systems, MARPOL Special Areas, RFMO temporal or spatial closed areas such as “Vulnerable Marine Ecosystems” (VMEs) or “other effective area-based conservation measures” (OECMs).
- Cross-sectoral ABMTs, including Marine Protected Areas (MPAs), Highly Protected Marine Areas (HPMAs) or marine spatial planning, requiring consultation, cooperation and coordination across multiple organizations and bodies to manage clearly defined geographical areas.
While the management objectives and approaches may differ, the development of a well-managed, representative and connected network of protected areas will support and build resilience to climate change. The OSPAR MPA network comprises 572 MPAs covering 490 552 km2 or 11% of the OSPAR Maritime Area. While all 12 Contracting Parties bordering the North-East Atlantic have designated MPAs, distribution is uneven across the five OSPAR Regions and the network cannot, as yet, be considered ecologically coherent (OSPAR, 2018).
Appropriate management is in place for some OSPAR MPAs, but many still await implementation. As a result, only 17% of OSPAR MPAs are known to be moving towards or have achieved their conservation objectives. A number of OSPAR countries also have area-based Other Effective Conservation Measures (OECMs) and non-OSPAR MPAs designated for specific features. These are protected, for the most part, through fisheries management measures (ICG-MPA 21/3/1.Add3).
A well-managed OSPAR MPA network will be crucial for maintaining protection of species and habitats and their resilience to the effects of climate change. There are two main areas where the MPA network may contribute to resilience. First, the protection of habitats and species across the MPA network at large spatial scales can contribute to ensuring that particular species or habitats survive within the network, even if they are lost in some locations due to changing ecological conditions or extreme events (such as increased frequency of storms or elevated sea surface temperature). Second, the MPA network can promote resilience to climate change by ensuring the maintenance and restoration of specific habitats that play vital roles in terms of climate regulation and regulation of ecosystem services. Such “blue carbon” habitats include kelp forests, seagrass beds, salt marshes or other habitats that represent effective carbon sinks or carbon. Other habitats such as reefs may provide valuable coastal ecosystem protection services. Such nature-based solutions also include providing protection from storm surges and regulating the effects of extreme weather events on coastal ecosystems and the communities they support.
The management of MPAs can be either active or passive. In active restoration, intervention is required so as to help restore ecosystems or increase the presence of species and features. Passive restoration allows ecosystems to recover via natural processes through the removal of pressures caused by human activities.
Three operational Objectives under OSPAR’s North-East Atlantic Environmental Strategy will assist Contracting Parties in developing area-based management tools and improving the resilience of ecosystems in the OSPAR Maritime Area. These are:
- S5.O1: By 2030, OSPAR will further develop its network of marine protected areas (MPAs) and other effective area-based conservation measures (OECMs) to cover at least 30% of the OSPAR Maritime Area to ensure it is representative, ecologically coherent and effectively managed to achieve its conservation objectives
- S5.O4: By 2025 at the latest, OSPAR will take appropriate actions to prevent or reduce pressures to enable the recovery of marine species and benthic and pelagic habitats in order to reach and maintain good environmental status as reflected in relevant OSPAR status assessments, with action by 2023 to halt the decline of marine birds
- S11.02: By 2023 and every six years thereafter, OSPAR will assess at a regional scale the OSPAR network of MPAs in respect of the resilience of marine biodiversity to climate change, with the aim of ensuring that the network provides a good representation of species and habitats and that its spatial design and management regime remains relevant
Ecosystem restoration and Resilience
Ecosystem restoration – the prevention, halting and reversal of the degradation of ecosystems – is one example of a nature-based solution. More and more, ecosystem restoration is also recognised for its role in increasing resilience to climate change, and thus in indirectly benefiting human wellbeing. On average, the benefits of ecosystem restoration are ten times higher than the costs, with benefits including: employment, business spending, gender equality, and local investment in education and livelihoods (Montanarella et al., 2018). The additional value to be obtained, through indirect benefits such as the climate resilience resulting from regulation services provided by the ocean, has still to be taken into account. Currently, the UN Decade on Ecosystem Restoration 2021-2030 is consolidating ecosystem restoration efforts globally in support of the UN’s sustainable development goals (SDGs).
Coastal marine restoration is still a relatively young field, although it has gained in popularity recently (Saunders et al., 2020). Marine restoration is still relatively less common practice than in terrestrial and freshwater systems. This may be due to the challenging environment (Saunders et al., 2020), limited information on baselines and longer time scales (Danovaro et al., 2021), or other technical, social and economic barriers (see for example Stewart-Sinclair et al., 2020).
The natural regeneration of over-exploited ecosystems through passive restoration can be a low-cost and effective tool when it comes to offshore waters and carbon-rich sediments supporting the ocean’s regulation function. Both passive and active restoration of marine ecosystems will contribute to higher ocean resilience to climate-induced changes and thus benefit nature and human society.
Natural capital approaches, where economic value is assigned to ecosystems and their components that provide valuable goods and services to society, are increasingly being recognised as a key tool to support ecosystem restoration (Farrell et al., 2021). Under NEAES 2030, OSPAR will “start accounting for ecosystem services and natural capital by making maximum use of existing frameworks in order to recognise, assess and consistently account for human activities and their consequences in the implementation of ecosystem-based management”.
Impact | Cumulative Effects |