Human activities exerting pressures on benthic habitats
Benthic habitats are impacted by activities operating and/or interacting with the biotic and abiotic components of the seafloor, sometimes over multiple spatial and temporal scales. The nature, periodicity and scale of these activities will determine the degree and extent of the impacts. The characteristics of key activities of particular relevance to benthic habitats are summarised in Table A.1 and the following sections.
Arctic Waters | Greater North Sea | Celtic Seas | Bay of Biscay and Iberian Coast | Wider Atlantic | |
---|---|---|---|---|---|
Fish and shellfish harvesting | |||||
Intensity | H | H | H | M | L |
Trend since QSR2010 | ↓ | ↑ | ↑ | ↔ | ↔ |
Trend to 2030 | ? | ? | ? | ? | ? |
Extraction of minerals | |||||
Intensity | L | H | M | M | L |
Trend since QSR2010 | ↔ | ↓ | ↔ | ↑ | ↔ |
Trend to 2030 | ? | ? | ? | ? | ? |
Agriculture | |||||
Intensity | L | H | M | M | L |
Trend since QSR2010 | ↔ | ↔ | ↔ | ↔ | ↔ |
Trend to 2030 | ↔ | ↔ | ↔ | ↔ | ↔ |
Aquaculture | |||||
Intensity | H | H | M | M | L |
Trend since QSR2010 | ↑ | ↑ | ↔ | ↑ | ↑ |
Trend to 2030 | ↑ | ↑ | ↑ | ↑ | ↑ |
Oil and gas | |||||
Intensity | M | H | M | L | L |
Trend since QSR2010 | ↔ | ↔ | ↔ | ↔ | ↔ |
Trend to 2030 | ↔ | ↔ | ↔ | ↔ | ↔ |
Renewable energy | |||||
Intensity | L | H | M | L | L |
Trend since QSR2010 | ↑ | ↑ | ↑ | ↑ | ↔ |
Trend to 2030 | ↑ | ↑ | ↑ | ↑ | ↔ |
Shipping | |||||
Intensity | M | H | H | H | L |
Trend since QSR2010 | ↔ | ↔ | ↔ | ↔ | ↔ |
Trend to 2030 | ? | ? | ? | ? | ? |
Tourism and leisure | |||||
Intensity | L | H | M | H | L |
Trend since QSR2010 | ↑ | ↑ | ↔ | ↑ | ↑ |
Trend to 2030 | ↑ | ↑ | ↔ | ↑ | ↑ |
The main anthropogenic activities interacting with benthic ecosystems are described in the following sections.
Fish and shellfish harvesting (professional, recreational) [Extraction of living resources]:
Human activities such as fishing can have adverse effects on marine environments and natural resources. For example, the effects of bottom trawling have the potential to cause physical disturbance to a broad variety of marine species and habitats (see: OSPAR Feeder Report 2021- Fisheries ). Pressures associated with bottom-contact fishing are categorised by métier-specific penetration depths, depending whether they cause surface or subsurface abrasion (JNCC, 2011; Church et al., 2016). Surface abrasion pressure can be caused by fishing gear, including trawling nets, and can damage benthic communities on the seafloor surface and upper layers of sediment (< 2 cm in depth) (ICES, 2021). Subsurface abrasion pressure can be caused by gear types including beam trawls and is defined as the penetration of substrate ≥ 2 cm below the surface (Church et al., 2016); it can potentially damage communities living within the sediment, such as burrowing bivalves.
Physical pressures from fishing can result in both permanent and potentially reversible changes to the original morphological state of the seabed (e.g., secondary succession and shifting baselines following trawl events). The degree of impact associated with fishing is conditioned by the type of fishing (e.g., the type of gears used in mobile fisheries) and the biological traits of the affected organisms, such as life stages and physical structure (Collie et al., 2001; Lambert et al., 2014; van Denderen et al., 2015). Various traits of marine species and habitats define their ability to tolerate or resist changes (resistance) and their ability to recover (resilience) (Tillin et al., 2010; BioConsult, 2013; Eno et al., 2013; Lambert et al., 2014; Tillin and Tyler-Walters, 2014a and 2014b; Tyler-Walters et al., 2018). For example, sessile, free-standing epibenthic organisms cannot move out of the way of trawl doors or towed nets and may thus sustain direct injuries and/or mortalities, indicating low resistance to the impact. (See: Extent of Physical Disturbance to Benthic Habitats: Fisheries with mobile bottom-contacting gears – Results – Figure 3 and Figure 4).
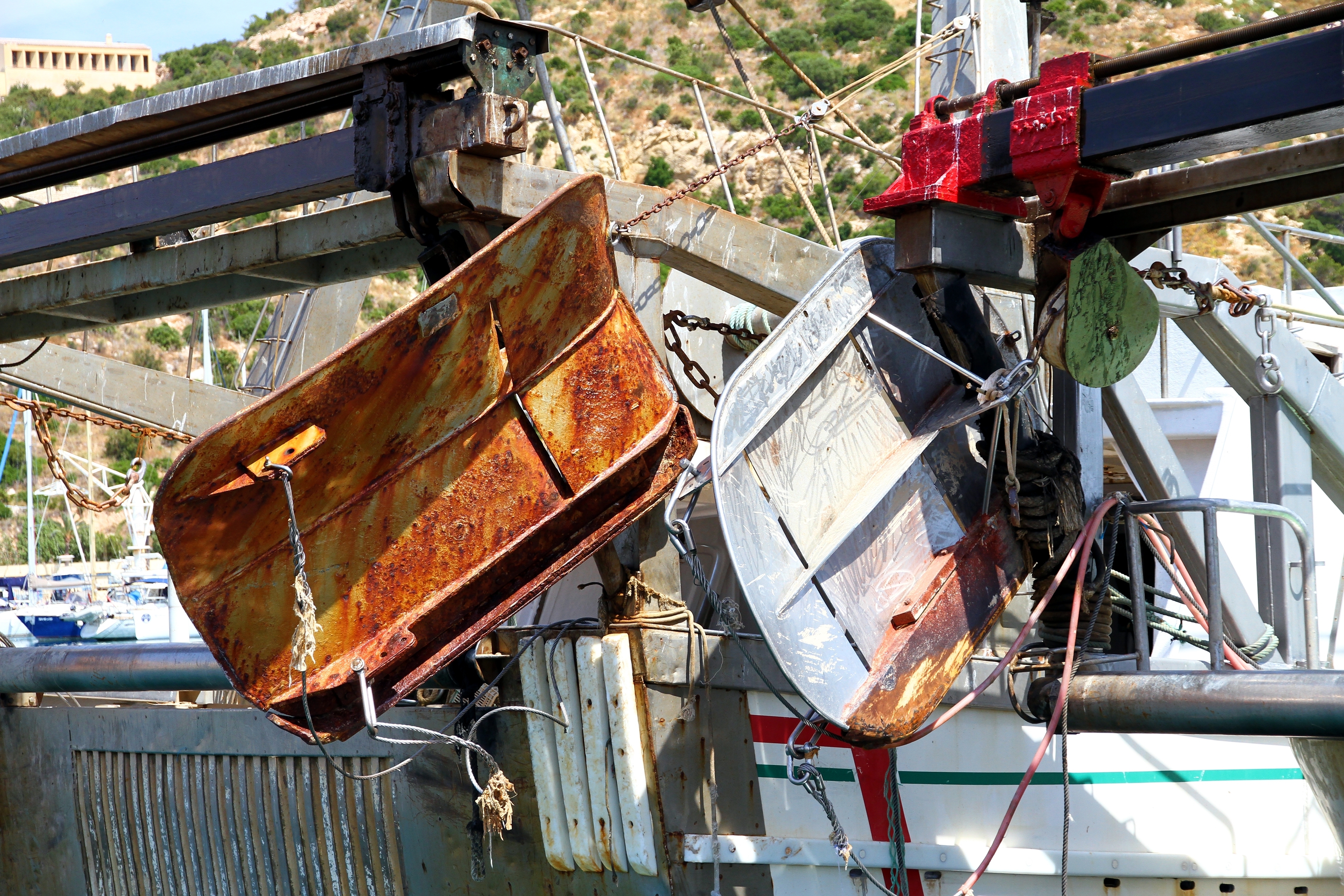
Sessile, free-standing epibenthic organisms cannot move out of the way of trawl doors and may thus sustain direct injuries and/or mortalities. © Shutterstock
Extraction of minerals - coastal and shelf (rock, metal ores, gravel, sand, shell) [Extraction of non-living resources]:
The extraction of materials from the seabed will directly impact benthic habitats in the relevant locations. The physical extraction of resources from the seafloor can have deleterious effects on benthic organisms and the structure of habitats, through the removal of substrate and the modification of the surrounding environment (Newell et al., 1998; Desprez, 2000; Newell and Woodcock, 2013). The nature and degree of the impact is dependent on the intensity of the activity and the associated method of extraction. The most common extraction method used in offshore marine environments is trailing suction hopper dredging, which creates shallow furrows that extend for several kilometres (initially 0,5 m deep and generally 2-3 m wide, depending on the type of resources being extracted) (Tillin et al., 2011; Last et al., 2011; Newell and Woodcock, 2013). The associated physical impacts can extend for many kilometres, potentially lowering the seabed by several metres through consistent and repeated dredging within a given location (Tillin et al., 2011; BMAPA and TCE, 2017). By contrast, the less common static suction dredging, or ‘anchor dredging’, can create deep (5-10 m) depressions in the seabed and can be used to target specific types of sediment (Tillin et al., 2011; Last et al., 2011; Newell and Woodcock, 2013). Dredging depressions can create morphological irregularities in the seabed, with infill and degradation rates varying according to local and regional hydrodynamic and sedimentation regimes (BMAPA and TCE, 2017). Monitoring suggests that trailer dredge furrows can degrade over durations of three to seven years following impact (dependent on initial and resulting sedimentology) (Cooper et al., 2005). By contrast deeper, more prominent depressions, often associated with static dredging, can degrade over longer time frames, sometimes resulting in a permanent lowering of the seabed (Cooper et al., 2007). (See: OSPAR Feeder Report 2021 – Extraction of non-living resources , Extent of Physical Disturbance to Benthic Habitats: Aggregate Extraction (BH3b), Pilot Assessment of Area of Habitat Loss (BH4))
Extraction of minerals - deep-sea mining [Extraction of non-living resources]:
Within the OSPAR Maritime Area, several zones have been identified as potential targets for future mineral exploration and exploitation (OSPAR, 2021). Deep seabed mining of seafloor mineral resources therefore has potential for development in the OSPAR Maritime Area, although concerns have been raised about the potential impacts on these poorly known and very sensitive deep-sea habitats owing to their stable environmental conditions and fragile natural biological communities.
The deep sea (> 200 m depth) contains highly specific and sensitive habitats, owing to environmental conditions characterised by very little exposure to natural disturbances and an absence of light. Direct mining in benthic ecosystems could cause notable local mortality and removal of inhabiting fauna, removal of substrata and habitat loss, habitat fragmentation and modification (i.e., change of mineral and sediment composition, topography, chemical regimes). Smothering or entombment of benthic fauna, clogging of suspension feeders or dilution of deposit feeders’ food resources could also occur (Sharma 2015, Gollner et al., 2017, 2022). Additionally, mining activities can promote the input of certain substances (such as the release of sediment-bound toxic metals into the water column and sediment) as well as an increase in anthropogenic inputs of energy, like sound and light (OSPAR, 2021). Mining activities of interest within the OSPAR Maritime Area might also overlap with rare and relatively understudied habitats, such as hydrothermal vents rich in polymetallic sulphides (Boschen et al., 2013), or biodiversity hotspots such as rock substrates with cobalt-rich ferromanganese crusts on seamounts (Gollner et al., 2017). Mining of these crusts typically occurs in hard rock habitats on seamounts which have a rich and specific biodiversity characterised by long-lived and slow-growing species (e.g., biogenic structures) and are thus highly sensitive and vulnerable.
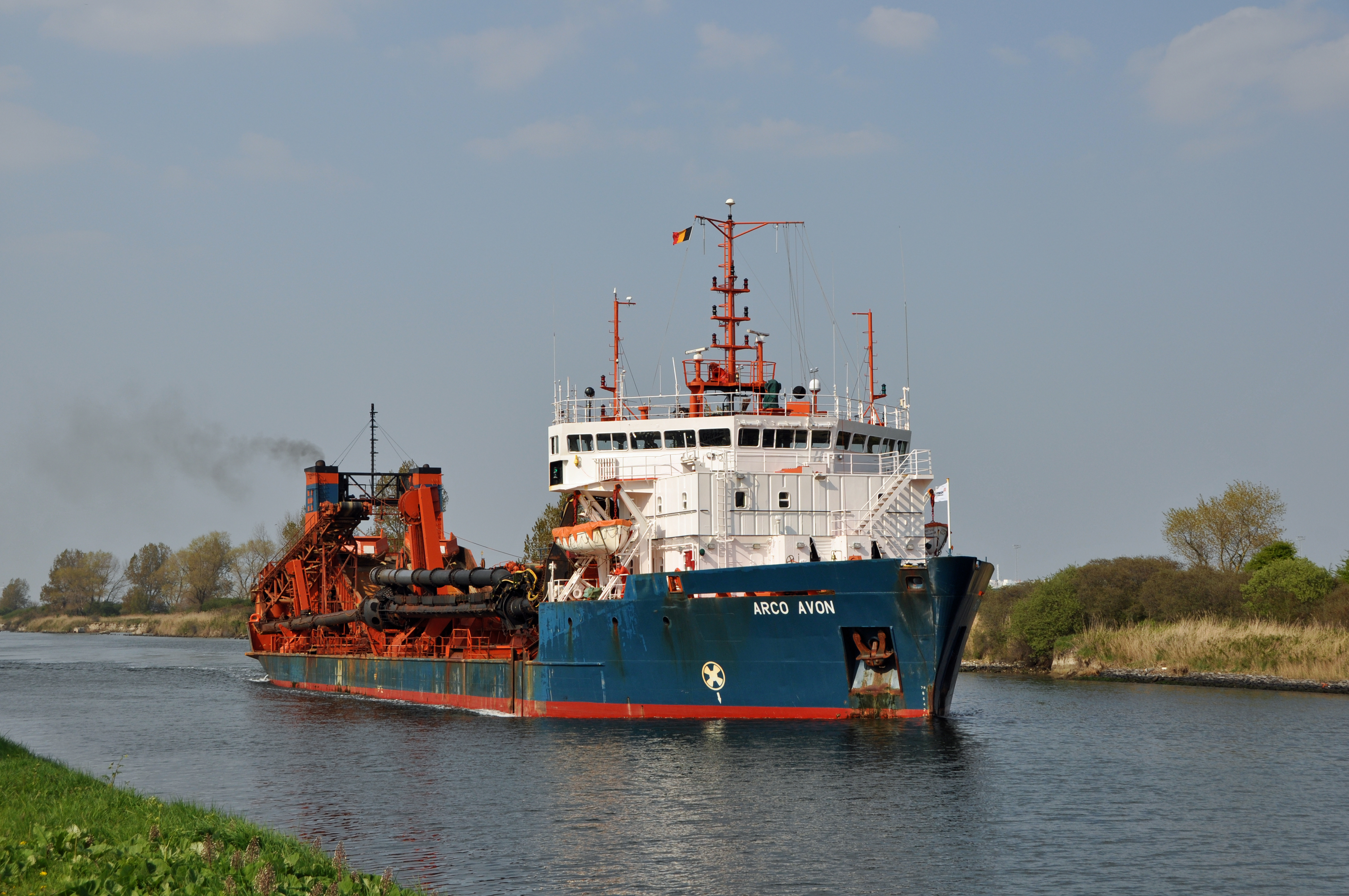
The British trailing suction hopper dredger Arco Avon in the Boudewijn canal (Belgium). © Marc Ryckaert
Restructuring of seabed morphology, including dredging and deposition of materials [Physical restructuring of rivers, coastline or seabed, notably for water management]:
The dredging and deposition of materials at sea, predominantly associated with the maintenance of navigable channels, will directly impact the exposed benthic habitats and their surrounding environment. Benthic communities are physically removed and/or covered by sediment, which often means a loss, or at least significant physical disturbance, of the impacted habitat. Several ongoing dredging works are associated with periodical maintenance activities at fixed disposal sites; they require preliminary and ongoing environmental impact assessment studies. As a consequence, habitat quality might be (semi) permanently in a poor condition, even though some examples exist of replacement by specific benthic communities which flourish under these conditions, such as on the 'artificial' steep walls associated with high water currents. Changing seabed morphology can have an impact on larger scale hydrodynamic conditions, with consequences for seabed shear-stress, sedimentation/erosion patterns and characteristics (type of sediment). In the case of several dredging and/or deposition sites, this can result in a pattern of interactions with substantial consequences. The risks of impacts on benthic habitats are higher in unnaturally confined areas like modified estuaries and inlets. Exposed ecosystems typically reach unbalanced or altered condition when dredging is deeper. The deposition of materials very different from the naturally occurring materials that are originally present typically leads to the highest impacts. (See: Assessment of Data on the Management of Wastes or Other Matter (Dredged Material) 2008 - 2020 ).
Tourism and leisure infrastructure [Tourism and leisure]:
Infrastructure related to tourism, such as marinas, piers, parking, beaches and coastal pathway facilities, can affect benthic habitats (‘loss and disturbance´) through the introduction of structures and increased human activities, and through the introduction of non-native species in some cases.
Tourism has shown a non-linear increase in the last century, especially since 1950 when there has been an exponential 28-fold rise in international tourism (Davenport and Davenport, 2006). According to Davenport and Davenport (2006) the greatest ecological threats from mass tourism are the infrastructure and transport arrangements required to support it. This can cause major impacts on marine habitats, notably through the development of marinas, associated coastal defences, the water treatment and desalination plants (including their waste product of highly concentrated brine) needed to supply fresh water to a growing population, and the associated increased inputs of organic and nutrient pollution.
Even some leisure activities which seem sustainable, such as sailing or diving, may have impacts on marine habitats when carried out in especially sensitive marine habitats. Diving in submarine caves can affect the distribution of benthic assemblages within them by reducing the abundance of erect growth form organisms (Guarnieri et al., 2012). Anchoring in sensitive marine biogenic habitats such as Zostera spp. or Posidonia oceanica seagrass beds can also have important negative impacts (Francour et al., 1999; Milazzo et al., 2004). In general, numbers of recreational boats, and their engine sizes, are increasing. This leads to negative environmental effects such as increased congestion, the development of piers, berths and floating bridges, and disturbance caused by higher speeds, swell and beach erosion. Additionally, most recreational boats directly emit exhaust fumes directly into the water which can contain a cocktail of toxic and environmentally harmful substances, including polycyclic aromatic hydrocarbons (PAHs), several of which are toxic, carcinogenic and / or mutagenic. The exhaust gases also contain soot, unburned oils and fuels, fertilising and acidifying nitrogen compounds as well as carbon monoxide and carbon dioxide. In areas with the highest leisure boat traffic, this has resulted in elevated PAH levels of in sediments, for example. In the West Coast region of Sweden there are approximately 110 000 leisure boats of all types (Swedish Transport Agency, 2021) many of them powered by gasoline or diesel fuels. The natural harbours on the Swedish coast are popular alternatives to urban harbours and marinas, as the legal right of public access makes it possible to anchor, moor and spend the night in a scenic archipelago, which leads to significant local physical stress for benthic habitats. (Nordberg et al., 2022). (See: OSPAR Feeder Report 2021 – Recreation and Tourism in the North-East Atlantic ).
Transmission of electricity and communications (cables) [Production of energy]:
The placement of cables for electricity and telecoms is driven by worldwide communications and energy transmission and is expected to increase due to predicted growth in the marine renewable energy sector. The cable installation, maintenance, and decommissioning phases introduce noise, pollution, turbidity and physical disturbance to the seafloor. Electromagnetic fields, heat emission, pollution and reef effects are associated with the operational life of the cable.
The majority of marine cables are buried to mitigate the risk of damage from fishing activities and anchoring. Impacts to benthic habitats and the seafloor include disturbance, sediment alteration, loss of habitat, and the introduction/spread of non-native species (introduction via maintenance vessels or spread via the installation of artificial substrate in the form of cables or cable protection). Other indirect effects include induced magnetism, noise, thermal radiation, or chemical and physical interactions between seawater and the cable insulation or protection layers (Vasilescu and Dinu, 2021). Disturbance to the seafloor is generally limited to the cable corridor (around 10 m) and is generally temporary. However, longer-term effects may also be experienced, with unburied cables acting as dragging features when shifted by tides and wave actions (Merck and Wasserthal, 2009). The impact of electromagnetic fields on the orientation and migratory habits of fish and surrounding benthic fauna is poorly understood (Copping et al., 2020).
Coastal defence and flood protection [Physical restructuring of rivers, coastline or seabed (water management)]:
Coastal defence and flood protection works include the construction, operation and decommissioning of, for example, seawalls, flood doors and embankments. Structures may be constructed on or adjacent to benthic habitats. Their installation can cause both direct and indirect impacts on benthic habitats (Martin et al., 2005), and is one of the main sources of habitat loss. When placed on soft sediments, coastal defences change the original benthic habitat by installing hard substrate, greatly changing the associated community. Furthermore, coastal defences produce important indirect effects by modifying the wave and deposition regime, altering sedimentation processes and potentially generating habitat loss away from the location of the activities. The effects of coastal defences in several European countries were analysed by Martin et al., in 2005. These authors identified a general trend across all locations and countries showing that changes in sediment and infauna associated with coastal defences seemed to be inevitable and usually induced negative changes, particularly on the landward side and in the presence of additional structures.
Renewable energy generation (wind, wave and tidal power), including infrastructure [Production of energy]:
Construction, operation, and decommissioning activities associated with renewable energy developments may directly interact with benthic habitats and/or may affect habitat conditions.
Offshore wind has proven to be a valuable source of renewable and clean energy. European seas host over 75% of the global capacity (GWEC, 2019), implying the widespread installation of infrastructure. This comprises multiple turbines (with their foundations) as well as complex systems of undersea cables and transformers for energy transmission and distribution (the latter occurring only on land). The majority of installations are found in the Greater North Sea Region– a global multi-source renewable energy hotspot. The first impact on the benthic habitat is caused by the sealing of the seafloor by benthic infrastructure, causing a loss of habitat (generally siliciclastic seafloor habitats are replaced by artificial substrates) (Galparsoro et al., 2021). Consequently, wind and tidal energy structures are responsible for altering the initial benthic community (Baker et al., 2020; Coolen et al., 2022) by introducing intertidal and subtidal artificial hard substrate habitats for epifaunal and colonising organisms (Degraer et al., 2020) and creating the potential for invasive species (De Mesel et al., 2015). The feeding activity of these newly introduced fauna may lead to habitat alterations through organic enrichment resulting from their filter-feeding and excretion behaviour (see e.g., Project OUTFLOW). This might be more prominent in the near field (i.e., the immediate vicinity of the foundations and turbines), while the extent of the far-field effects will be linked to the local hydrodynamics, although this remains poorly studied and thus poorly understood. The potential for large-scale (i.e., basin-scale) habitat alteration is significant given the forecast increase in offshore infrastructural development involved in the decarbonisation of energy production in Europe. (See: OSPAR Feeder Report 2021 - Offshore Renewable Energy Generation ).
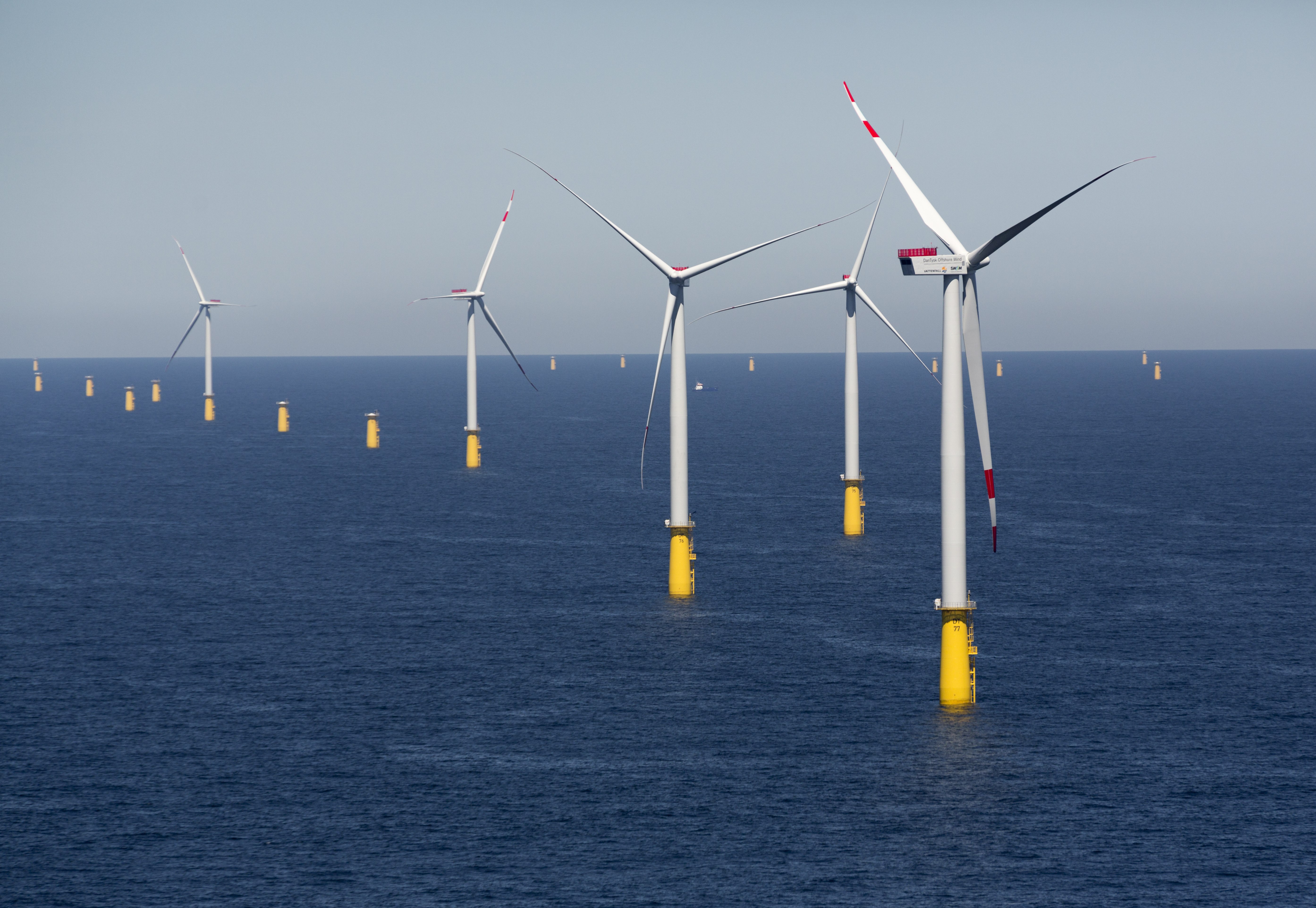
© Paul Langrock
Extraction of oil and gas, including infrastructure [Extraction of non-living resources]:
The need for energy security has meant that oil and gas development has remained constant in most of the OSPAR Maritime Area, although there are decreases in some parts. Most installations are located in the Greater North Sea, followed by the Arctic Waters (south of the ice edge) and Celtic Seas. Exploration, operation, decommissioning and the associated infrastructure can directly interact with benthic habitats. Physical impacts result from the placement of installations, pipelines and associated structures. The sealing of the seabed leads to loss of soft sediment habitats. Changes of the physical habitat also occur due to scouring or protections around structures. The physical presence of installations leads to changes of benthic community structures, notably favouring species associated with hard substrates. Epibenthic filter feeders settle on platform legs, while suspension-feeding organisms in particular prevail on the surrounding seabed. These notably benefit from the organic material provided by other species colonising the foundation structures. As a consequence, related benthic predators also increase and contribute to changes in the local food web.
Operational platforms impact benthic organisms through discharges of produced water, oil, chemicals and drill cuttings. The drilling process involves the discharge of drilling fluids along with drill cuttings, which may cause smothering in the near vicinity of the well location. The contamination results in lower diversity and abundance of benthic organisms and an increased dominance of tolerant opportunistic species for several square kilometres around the well location. (See: Assessment of impacts of the offshore oil and gas industry on the marine environment ).
Marine aquaculture [Cultivation of living resources]:
This activity may affect benthic habitats through placement of structures (physical), nutrient and organic enrichment (physical and chemical inputs), and also as one of the main vectors for the introduction of non-indigenous species (biological pressure). This type of activity has increased very rapidly over recent decades, alongside a diversification of techniques and the addition of new farmed species, which could lead in turn to wider and sometimes negative impacts on ecosystem services (Gentry et al., 2022; Naylor et al., 2021). The impacts on benthic habitats are often very locally documented but the few reviews conducted at wider scales highlight both direct and indirect effects on benthic communities and habitats (McKindsey et al., 2011), notably in intertidal or estuarine areas (Simenstad and Fresh, 1995). (See: OSPAR Feeder Report 2021 - Aquaculture, Human Activities Thematic Assessment)
Agriculture [Cultivation of living resources]:
Agriculture has been an important social and economic feature of European societies since prehistoric times. Nitrogen pollution of water has increased greatly from various sources, notably the large amounts of mineral fertilisers applied since the 1950s to boost agricultural food and feed production (Marshall Plan of 1947; Common Agricultural Policy (CAP) of 1957, which entered into practice in 1962; FAO, 2018), resulting in intensification and specialisation of agriculture (JRC, 2022). The scientific literature dealing with the land-to-sea continuum integrating diffuse nutrient loading from agricultural activities into the watershed, including their effects on the coastal zone, describes these various aspects and their related impacts (e.g., Blann et al., 2009; Fierro et al., 2021). Inputs of nutrients and organic matter from agriculture may lead to eutrophication of benthic habitats, particularly those in coastal areas or under direct influence from freshwater inputs. (See: OSPAR Feeder Report 2021 - Agriculture , Human Activities Thematic Assessment ).
Urban uses, Industrial uses, Waste treatment and disposal [Urban and industrial uses]:
Clean water supply and waste water treatment have required huge investment across Europe in recent decades. Much has been done to provide for the collection and treatment of urban waste water, but new pressures (such as adapting to climate change, providing facilities in urban and rural areas and tackling newly identified pollutants) require substantial investment over and above the maintenance of the existing infrastructure (EEA, 2021). The European Union Water Framework Directive (EU WFD) (2000/60/EC) and all the national programmes related to water quality have established a framework for the protection of rivers, lakes, transitional waters (estuaries), coastal waters and groundwater. The EUWFD aims to ensure that all surface water bodies achieve good chemical and ecological status and display minimal signs of impact from human development. The requirements of the EU WFD and any related national programmes constitute the baseline for managing water pollution coming from urban areas. Benthic habitats exposed to urban and industrial contaminated waste waters are affected by various pollutants (e.g., nutrient and organic enrichments, contaminants, microbial pathogens), which are described in other dedicated thematic assessments. (See: OSPAR Feeder Report 2021 – Waste water , Human Activities Thematic Assessment , Eutrophication Thematic Assessment and Hazardous Substances Thematic Assessment ).
Transport infrastructure [Transport]:
Transport infrastructure, such as ports or any related facility, can affect benthic habitats through pressures resulting from the construction and operational phase of associated structures. Environmental alteration or the replacement of benthic habitat by constructed infrastructure leads to a reduction in ecosystem functioning. Marine artificial infrastructure is progressively colonised by biota; however, this infrastructure does not function as an alternative to a natural hard substrate. The introduction of hard infrastructure along a sedimentary coastline can modify local biodiversity patterns and facilitate the establishment of non-indigenous species (Bacchiocchi and Airoldi, 2003). The alteration of a naturally sloping habitat to a general vertical habitat can have several adverse effects, such as modified distribution of plants and biota, food web interactions, and abnormal densities of organisms, which may result in turn in reduced size or reproductive output (Bulleri and Chapman, 2010). The addition of infrastructure often also leads to natural habitat fragmentation which reduces dispersal and movement across the landscape, resulting in a reduction of unique taxa and species variability (Goodsell, Chapman and Underwood, 2007). (See: Human Activities Thematic Assessment ).
Fish and shellfish harvesting (professional, recreational) [Extraction of living resources]:
BioConsult, 2013. Seafloor integrity - Physical damage, having regard to substrate characteristics (Descriptor 6). A conceptual approach for the assessment of indicator 6.1.2: ‘Extent of the seafloor significantly affected by human activities for the different substrate types. Report within the R & D project ‘Compilation and assessment of selected anthropogenic pressures in the context of the Marine Strategy Framework Directive’, UFOPLAN 3710 25 206. http://www.bioconsult.de/veroeffentlichungen/BioConsult_FinalDraft_Indicator6.1.2.pdf
Church N.J., Carter A.J., Tobin D., Edwards D., Eassom A., Cameron A., Johnson G.E., Robson, L.M. and Webb K.E. 2016. JNCC Recommended Pressure Mapping Methodology 1. Abrasion: Methods paper for creating a geo-data layer for the pressure ‘Physical Damage (Reversible Change) - Penetration and / or disturbance of the substrate below the surface of the seabed, including abrasion’. JNCC report No. 515, JNCC, Peterborough. https://data.jncc.gov.uk/data/5874e65d-324b-4f6b-bce2-bfc7aab5ba7f/JNCC-Report-515-FINAL-WEB.pdf
Collie, J. S., Hall., S. J., Kaiser, M. J., and Poiner, I. R. 2001. A quantitative analysis of fishing impacts on shelf-sea benthos. Journal of Animal Ecology, 69(5); 7785-798.
Eno, N.C., Frid, C. L. J., Hall, K., Ramsay, K., Sharp, R. A. M., Brazier, D. P., Hearn, S., Dernie, K. M., Robinson, K. A, Paramor, O. A. L. and Robinson, L. A. 2013. Assessing the sensitivity of habitats to fishing: from seabed maps to sensitivity maps. Journal of Fish Biology, 83, 826-846.
ICES, 2021. OSPAR request on the production of spatial data layers of fishing intensity / pressure. ICES Technical service. https://ices-library.figshare.com/articles/report/OSPAR_request_on_the_production_of_spatial_data_layers_of_fishing_intensity_pressure/18639182
JNCC, 2011. Review of methods for mapping anthropogenic pressures in UK waters in support of the Marine Biodiversity Monitoring R&D Programme. Briefing paper to UKMMAS evidence groups. Presented 06/10/2011. Available on request from https://jncc.gov.uk/contact/
Lambert, G. I., Jennings, S., Kaiser, M. J., Davies, T. W. and Hiddink, J. G. 2014. Quantifying recovery rates and resilience of seabed habitats impacted by bottom fishing. Journal of Applied Ecology, 51(5); 1326-1336.
OSPAR, 2017. OSPAR Intermediate Assessment 2017. OSPAR Commission. Available at: https://oap.ospar.org/en/ospar-assessments/intermediate-assessment-2017/
Tillin, H.M., Hull, S.C. and Tyler-Walters, H. 2010. Development of a Sensitivity Matrix (pressures-MCZ / MPA features). Defra Contract No. MB0102 Task 3A, Report No. 22. https://www.marlin.ac.uk/assets/pdf/MB0102_Task3-PublishedReport.pdf
Tillin, H., Tyler-Walters, H., 2014a. Assessing the sensitivity of subtidal sedimentary habitats to pressures associated with marine activities. Phase 1 Report: Rationale and proposed ecological groupings for Level 5 biotopes against which sensitivity assessments would be best undertaken. JNCC Report No. 512A, 68 pp. https://hub.jncc.gov.uk/assets/4204b127-cda2-418c-abd1-ba3589341535
Tillin, H. & Tyler-Walters, H., 2014b. Assessing the sensitivity of subtidal sedimentary habitats to pressures associated with marine activities. Phase 2 Report – Literature review and sensitivity assessments for ecological groups for circalittoral and offshore Level 5 biotopes. JNCC Report No. 512B, 260 pp. https://hub.jncc.gov.uk/assets/742cd48d-4349-4894-bbbf-d38b1c158a1c
Tyler-Walters, H., Tillin, H.M., d’Avack, E.A.S., Perry, F. and Stamp, T. 2018. Marine Evidence based Sensitivity Assessment (MarESA) – A Guide. Plymouth: Marine Biological Association. https://www.marlin.ac.uk/assets/pdf/MarESA-Sensitivity-Assessment-Guidance-Rpt-Mar2018v2.pdf
Van Denderen, P. D., Bolam, S. G., Hiddink, J. G., Jennings, S., Kenny, A., Rijnsdorp, A. D. and van Kooten, T. 2015. Similar effects of bottom trawling and natural disturbance on composition and function of benthic communities across habitats. Marine Ecology Progress Series, 341, 31-43.
Extraction of minerals - coastal and shelf (rock, metal ores, gravel, sand, shell) [Extraction of non-living resources]:
British Marine Aggregate Producers Association (BMAPA) and The Crown Estate (TCE). (2017). The impacts of marine aggregate dredging. Good Practice Guidance: Extraction by Dredging of Aggregates from England’s Seabed. 9-10. [Online] Available at: https://www.bmapa.org/documents/BMAPA_TCE_Good_Practice_Guidance_04.2017.pdf (Accessed April 2021).
Cooper K.M., Eggleton J.D., Vize S.J., Vanstaen K., Smith R., Boyd S.E., Ware S., Morris C.D., Curtis, M. l., Limpenny D.S. and Meadows W.J. (2005). Assessment of the rehabilitation of the seabed following marine aggregate dredging-part II. Cefas Science Series Technical Report No. 130. Cefas Lowestoft. 82. https://www.marinedataexchange.co.uk/details/1179/2005-cefas-assessment-of-the-rehabilitation-of-the-seabed-following-marine-aggregate-dredging---part-ii/summary https://www.marinedataexchange.co.uk/details/1179/2005-cefas-assessment-of-the-rehabilitation-of-the-seabed-following-marine-aggregate-dredging---part-ii/summary
Cooper K., Boyd S., Eggleton J., Limpenny D., Rees H., and Vanstaen K. (2007). Recovery of the seabed following marine aggregate dredging on the Hastings Shingle Bank off the south-east coast of England. Estuarine Coastal and Shelf Science, 75(4), 547-558.
Desprez, M., 2000. Physical and biological impact of marine aggregate extraction along the French coast of the Eastern English Channel: short-and long-term post-dredging restoration. ICES journal of marine science, 57(5), pp.1428-1438. https://doi.org/10.1006/jmsc.2000.0926
Last, K. S., Hendrick, V. J., Beveridge, C. M., and Davies, A. J. (2011). Measuring the effects of suspended particulate matter and smothering on the behaviour, growth and survival of key species found in areas associated with aggregate dredging. pp. 70. https://portal.medin.org.uk/portal/start.php?tpc=015_04ca2d19d7045b0ba4f20b72fbf69fd4
Newell, R. C., Seiderer, L. J. and Hitchcock, D. R. (1998). The impact of dredging works on coastal waters: A review of the sensitivity to disturbance and subsequent recovery of biological resources on the seabed. Oceanography and Marine Biology. 36, 127-78.
Newell, R. C. and Woodcock, T. A. (2013). Aggregate dredging and the marine environment: an overview of recent research and current industry practice. The Crown Estate. 165pp. ISBN: 978-1-906410-41-4.
Tillin, H. M., Houghton, J. Saunders, E., Drabble, R. and Hull, S. C. (2011). Direct and Indirect Impacts of Aggregate Dredging. Science Monograph Series No. 1. Marine ASLF. 41pp. ISBN: 978 0 907545 43 9.
Extraction of minerals - deep-sea mining [Extraction of non-living resources]:
Boschen, R.E., Rowden, A.A., Clark, M.R. and Gardner, J.P. 2013. Mining of deep-sea seafloor massive sulfides: a review of the deposits, their benthic communities, impacts from mining, regulatory frameworks and management strategies. Ocean & coastal management, 84, pp.54-67.
Gollner, S., Kaiser, S., Menzel, L., Jones, D.O., Brown, A., Mestre, N.C., Van Oevelen, D., Menot, L., Colaço, A., Canals, M. and Cuvelier, D. 2017. Resilience of benthic deep-sea fauna to mining activities. Marine Environmental Research, 129, pp.76-101.
Gollner, S., Haeckel, M., Janssen, F., Lefaible, N., Molari, M., Papadopoulou, S., Reichart, G.J., Trabucho Alexandre, J., Vink, A. and Vanreusel, A. 2022. Restoration experiments in polymetallic nodule areas. Integrated Environmental Assessment and Management, 18(3), pp.682-696.
OSPAR Commission, 2021. Technical report on current understanding of deep seabed mining resources, technology, potential impacts and regulation along with the current global demand for minerals. OSPAR Commission. Publication Number: 790/2021. Available at: https://www.ospar.org/documents?v=46926
Sharma, R., 2015. Environmental issues of deep-sea mining. Procedia Earth and Planetary Science, 11, pp.204-211.
Tourism and leisure infrastructure [Tourism and leisure]:
Davenport, J. and Davenport, J. L. (2006). The impact of tourism and personal leisure transport on coastal environments: a review. Estuarine, coastal and shelf science, 67(1-2), 280-292.
Francour, P., Ganteaume, A. and Poulain, M. (1999). Effects of boat anchoring in Posidonia oceanica seagrass beds in the Port‐Cros National Park (north‐western Mediterranean Sea). Aquatic conservation: marine and freshwater ecosystems, 9(4), 391-400.
Guarnieri, G., Terlizzi, A., Bevilacqua, S. and Fraschetti, S. (2012). Increasing heterogeneity of sensitive assemblages as a consequence of human impact in submarine caves. Marine Biology, 159(5), 1155-1164.
Milazzo, M., Badalamenti, F., Ceccherelli, G. and Chemello, R. (2004). Boat anchoring on Posidonia oceanica beds in a marine protected area (Italy, western Mediterranean): effect of anchor types in different anchoring stages. Journal of Experimental Marine Biology and Ecology, 299(1), 51-62.
Nordberg, K., Björk, G., Lundin, L., Abrahamsson, K., Josefsson, S., Dahlberg, C. and Zar, I. (2022). Fritidsbåtars avgasutsläpp i skärgårdsmiljön (No. 2022:2). Havsmiljöinstitutet/Swedish Institute for the Marine Environment. In Swedish with english summary. Available at: https://havsmiljoinstitutet.se/sites/default/files/2022-09/fritidsbatars_avgasutslapp_i_skargarden_dr2.pdf
Swedish Transport Agency (Transportstyrelsen). (2021) Båtlivsundersökning. En undersökning om båtlivet i Sverige. Swedish Transport Agency Avdelning Sjö- och luftfart. Dnr 2021-2170. 108pp. accessed via https://www.transportstyrelsen.se/globalassets/global/sjofart/dokument/fritidsbatar1/transportstyrelsen-batlivsundersokningen-2020.pdf
Transmission of electricity and communications (cables) [Production of energy]:
Copping, A.E., Hemery, L.G., Overhus, D.M., Garavelli, L., Freeman, M.C., Whiting, J.M., Gorton, A.M., Farr, H.K., Rose, D.J. and Tugade, L.G. (2020) ‘Potential Environmental Effects of Marine Renewable Energy Development—The State of the Science’, Journal of Marine Science and Engineering, 8(11), p. 879. Available at: https://doi.org/10.3390/jmse8110879
Merck, T. and Wasserthal, R. (2009) ‘Assessment of the environmental impacts of cables’, OSPAR Biodivers. Ser., 12, p. 437.
Vasilescu, V.-F. and Dinu, D. (2021) ‘INSTALLATION OF SUBMARINE CABLES IN THE OFFSHORE WIND INDUSTRY AND THEIR IMPACT ON THE MARINE ENVIRONMENT’, Journal of marine Technology and Environment, 1(2021), pp. 43–51. Available at: https://doi.org/10.53464/JMTE.01.2021.07
Coastal defence and flood protection [Physical restructuring of rivers, coastline or seabed (water management)]:
Martin, D., Bertasi, F., Colangelo, M. A., de Vries, M., Frost, M., Hawkins, S. J. and Ceccherelli, V. U. (2005). Ecological impact of coastal defence structures on sediment and mobile fauna: evaluating and forecasting consequences of unavoidable modifications of native habitats. Coastal engineering, 52(10-11), 1027-1051.
Renewable energy generation (wind, wave and tidal power), including infrastructure [Production of energy]:
Baker, A. L., Craighead, R. M., Jarvis, E. J., Stenton, H. C., Angeloudis, A., Mackie, L. and Hill, J. (2020). Modelling the impact of tidal range energy on species communities. Ocean & coastal management, 193, 105221.
Coolen, J. W., Vanaverbeke, J., Dannheim, J., Garcia, C., Birchenough, S. N., Krone, R. and Beermann, J. (2022). Generalized changes of benthic communities after construction of wind farms in the southern North Sea. Journal of Environmental Management, 315, 115173.
Degraer, S., D.A. Carey, J.W.P. Coolen, Z.L. Hutchison, F. Kerckhof, B. Rumes, and J. Vanaverbeke. 2020. Offshore wind farm artificial reefs affect ecosystem structure and functioning: A synthesis. Oceanography 33(4):48–57, https://doi.org/ 10.5670/ oceanog.2020.405
De Mesel, I., F. Kerckhof, A. Norro, B. Rumes, and S. Degraer. 2015. Succession and seasonal dynamics of the epifauna community on offshore wind farm foundations and their role as stepping stones for non-indigenous species. Hydrobiologia 756:37–50, https://doi.org/10.1007/s10750-014-2157-1
Galparsoro, I., Korta, M., Subirana, I., Borja, Á., Menchaca, I., Solaun, O. and Bald, J. (2021). A new framework and tool for ecological risk assessment of wave energy converters projects. Renewable and Sustainable Energy Reviews, 151, 111539.
GWEC (Global Wind Energy Council). 2019. Global Wind Report 2018. Global Wind Energy Council, Brussels, Belgium, 62 pp.
Marine aquaculture [Cultivation of living resources]:
Gentry, Rebecca R., Heidi K. Alleway, Melanie J. Bishop, Chris L. Gillies, Tiffany J. Waters and Robert C. Jones. 2019. Exploring the potential for marine aquaculture to contribute to ecosystem services. Reviews in Aquaculture (2019). 12, 499–512. https://doi.org/10.1111/raq.12328
McKindsey, Christopher W., Archambault, Philippe, Callier, Myriam D., and Olivier, Frédéric. 2011. Influence of suspended and off-bottom mussel culture on the sea bottom and benthic habitats: a review. Canadian Journal of Zoology. 89(7): 622-646. https://doi.org/10.1139/z11-037
Naylor, R.L., Hardy, R.W., Buschmann, A.H. et al., 2021. A 20-year retrospective review of global aquaculture. Nature 591, 551–563 (2021). https://doi.org/10.1038/s41586-021-03308-6
Simenstad, C.A. and Fresh, K.L. 1995. Influence of intertidal aquaculture on benthic communities in Pacific Northwest estuaries: Scales of disturbance. Estuaries 18, 43–70 (1995). https://doi.org/10.2307/1352282
Agriculture [Cultivation of living resources]:
Blann, K. L., Anderson, J. L., Sands, G. R. and Vondracek, B. 2009. Effects of Agricultural Drainage on Aquatic Ecosystems: A Review. Critical Reviews in Environmental Science and Technology, 39:11, 909-1001, https://doi.org/10.1080/10643380801977966
FAO, 2018. More people, more food, worse water? A global review of water pollution from agriculture. Edited by Javier Mateo-Sagasta (IWMI), Sara Marjani Zadeh (FAO) and Hugh Turral. Published by the Food and Agriculture Organization of the United Nations, Rome, 2018 and the International Water Management Institute on behalf of the Water Land and Ecosystems research program of the CGIAR Colombo, 2018. ISBN 978-92-5-130729-8 (FAO). https://www.unwater.org/news/water-pollution-agriculture-global-review
Fierro, P., Valdovinos, C., Lara, C. and Saldías, G.S. 2021. Influence of Intensive Agriculture on Benthic Macroinvertebrate Assemblages and Water Quality in the Aconcagua River Basin (Central Chile). Water 2021, 13, 492. https://doi.org/10.3390/w13040492
JRC, 2022. Agriculture and coastal eutrophication across Europe. European Commission EU Science hub, Knowledge Hub on Water and Agriculture. Online web page accessed 6/11/2022: https://water.jrc.ec.europa.eu/eutrophication.html
Urban uses, Industrial uses, Waste treatment and disposal [Urban and industrial uses]:
EEA, 2021. Urban waste water treatment for 21st century challenges. European Environmental Agency Publications. Online webpage accessed 06/11/2022. https://www.eea.europa.eu/publications/urban-waste-water-treatment-for/urban-waste-water-treatment
Transport infrastructure [Transport]:
Bacchiocchi, F. and Airoldi, L. (2003) ‘Distribution and dynamics of epibiota on hard structures for coastal protection’, Estuarine, Coastal and Shelf Science, 56(5–6), pp. 1157–1166. Available at: https://doi.org/10.1016/S0272-7714(02)00322-0
Bulleri, F. and Chapman, M.G. (2010) ‘The introduction of coastal infrastructure as a driver of change in marine environments’, Journal of Applied Ecology, 47(1), pp. 26–35. Available at: https://doi.org/10.1111/j.1365-2664.2009.01751.x
Goodsell, P., Chapman, M. and Underwood, A. (2007) ‘Differences between biota in anthropogenically fragmented habitats and in naturally patchy habitats’, Marine Ecology Progress Series, 351, pp. 15–23. Available at: https://doi.org/10.3354/meps07144
Drivers | Pressures |