Pressures affecting benthic habitats
Impacts on benthic habitats and seafloor integrity are caused by several anthropogenic pressures. In the past few years, a great deal of attention has been given to pressures that cause seafloor abrasion and habitat loss, as well as those causing eutrophication and nutrient enrichment. The effects of these pressures on seafloor habitats are currently being assessed through a set of benthic indicators, although these are subject to further development. However, other pressures are also important, in particular inputs of heavy metals and contaminants, the extraction of living resources, the impact of introduced species, litter on the seafloor, and input from some forms of energy, such as thermal energy. Pressures from climate drivers and ocean acidification, such as increased coastal erosion or decreases in pH levels, are also a major driver of changes. The currently available evidence on the effects of these pressures is included in the Climate Change section of this assessment.
Physical disturbance to seabed (temporary or reversible) [Physical]:
Physical disturbance is understood as a change to the seabed from which it can recover, if the activity causing the disturbance ceases. The recovery time from physical disturbance should last, or is expected to last, for less than 12 years in order for the disturbance to be considered "temporary or reversible" (European Commission Decision 848/2017).
The extraction of living and non-living resources can result in interactions with the seabed and benthic habitats. Fish and shellfish harvesting are driven by the need for food security; they cover all aspects of catching wild fish and shellfish, whether with active or static gear or by hand gathering. Fishing gear such as trawls and dredges that drag across or penetrate the seabed can alter the seabed topography (e.g., scouring/scarring of sediments) and have major impacts on benthic communities. Bottom trawling leads to a decline of long-lived and large fragile species, whereas small and opportunistic species as well as scavengers are favoured. The persistence of such changes may depend on the gear type and prevailing site conditions (e.g., sheltered vs. exposed). Driven by construction and the demand for materials, the extraction of minerals (rock, metal ores, gravel, sand, shell) may also cause alterations to the seabed topography, changes in sediment composition, the removal of organisms and other temporary disturbance to benthic habitats, for example by mobilising and suspending sediments, thereby affecting water clarity and reducing the capability of benthic habitats to act as natural carbon sinks. See: OSPAR Indicator Assessments:
Physical loss (due to permanent change of seabed substrate or morphology and to extraction of seabed substrate) [Physical]:
Physical loss is defined as a permanent change to the seabed which has lasted or is expected to last for 12 years or more (European Commission Decision 848/2017). It Includes permanent habitat change (e.g., from input of hard substrate). The term "permanent change" of the seabed also covers instances in which recovery is only possible by active human intervention (e.g., coral, seagrass and kelp transplantations, removal of artificial structures, sand capping). The introduction of infrastructure to the marine environment for the purposes of coastal defence and flood protection, renewable energy generation, oil and gas extraction, tourism and leisure, and telecoms (cables) is driven by, respectively, the need for climate change mitigation, energy security, societal wellbeing, the creation of a digital society, and globalisation (telecoms). When infrastructure is introduced to the seabed, the result can be loss of the existing benthic habitat where the structures are present and the introduction of hard substrate to the location. There may also be subsequent indirect loss of benthic habitat (e.g., the introduction of hard seawalls prevents landward migration of intertidal habitats as sea levels rise). Direct benthic habitat loss may also result from the direct removal of seabed materials in order to extract minerals (e.g., sand and gravel extraction driven by construction and the need for materials) and from dredging and disposal (e.g., removal of sediments for navigational purposes, driven by trade and transport). These activities could also be classified as physical disturbance, depending on the duration of effects. Permanent changes of physical habitat caused by human activities (e.g., bottom trawling, aggregate extraction) or indirectly through the placement of man-made structures (e.g., hydrological changes) may also lead to physical loss of habitat types.
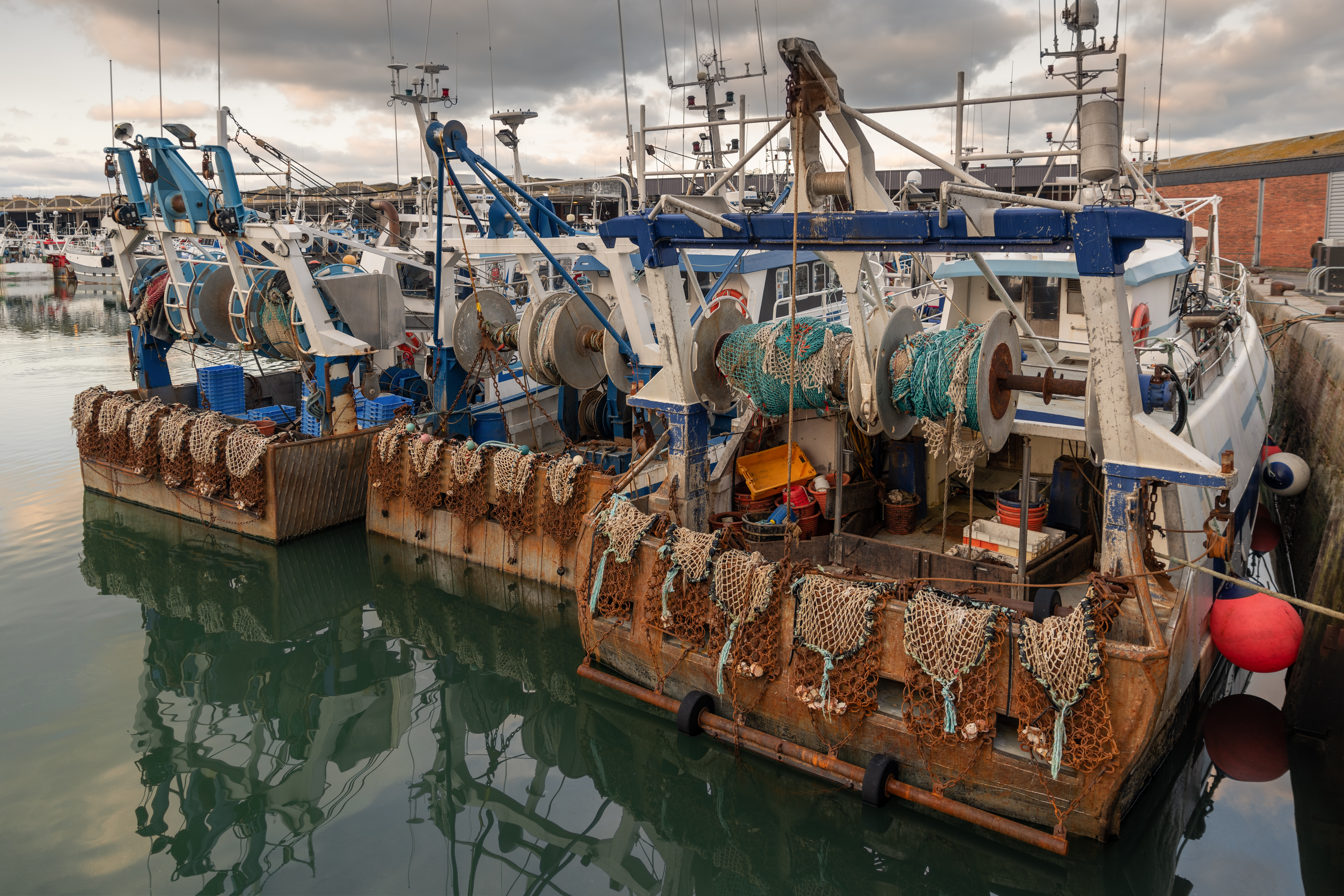
Fishing gear such as scallop dredges drag across or penetrate the seabed and alter the seabed topography. © Shutterstock
Input of nutrients - diffuse sources, point sources, atmospheric deposition, Input of organic matter - diffuse sources and point sources [Substances, litter and energy]:
Nutrient or organic enrichments may modify the growth, abundance and dynamics of plankton, algae and higher forms of plant life, which in turn may lead to a range of undesirable disturbances in the marine ecosystem including food webs. These can include oxygen depletion in bottom waters caused by the indirect death of impacted benthic species or significant shifts in the composition of the flora and fauna affecting both habitats and biodiversity. Several coastal waters of OSPAR Contracting Parties have, to a varying degree, been affected directly or indirectly by nutrient and / or organic enrichment due mainly to agriculture, urban and industrial waste waters and (more locally) marine culture. These can result in harmful or toxic algal blooms, change or loss of benthic vegetation owing to shading and competition for nutrients, or a drastic modification of benthic fauna communities (and associated functional aspects) due to specific sensitivities and population dynamics.
Pressures not currently and directly assessed by OSPAR benthic habitats indicators
Input of other substances [Substances, litter, and energy]:
Human activities can introduce a range of hazardous substances. This pressure type relates to increasing levels of contaminants in sediment or biota (e.g., PBDE, PAH, PCB, Metals, organotins) which can lead to changes in exposed benthic habitats and communities through their effects on differential mortalities, reduced breeding success or species fitness. ( See: Hazardous Substances Thematic Assessment )
Focus on the heavy metals in fish, shellfish and sediment:
Metals are ubiquitous hazardous substances that occur naturally in the environment. In marine sediments, metals are a natural part of different minerals and can be slightly more prevalent for some habitat types. Metals can be found in sediment, shellfish, and fish in all OSPAR Regions. The most documented toxic metals for humans and animals are mercury, cadmium and lead, known as “heavy or trace” metals, which enter the marine environment from a variety of sources. Direct inputs of metals used as antifouling chemicals (mainly copper) and corrosion anodes (mainly zinc) cause hot spots of metal concentrations in and around harbours, marine installations and along shipping routes.
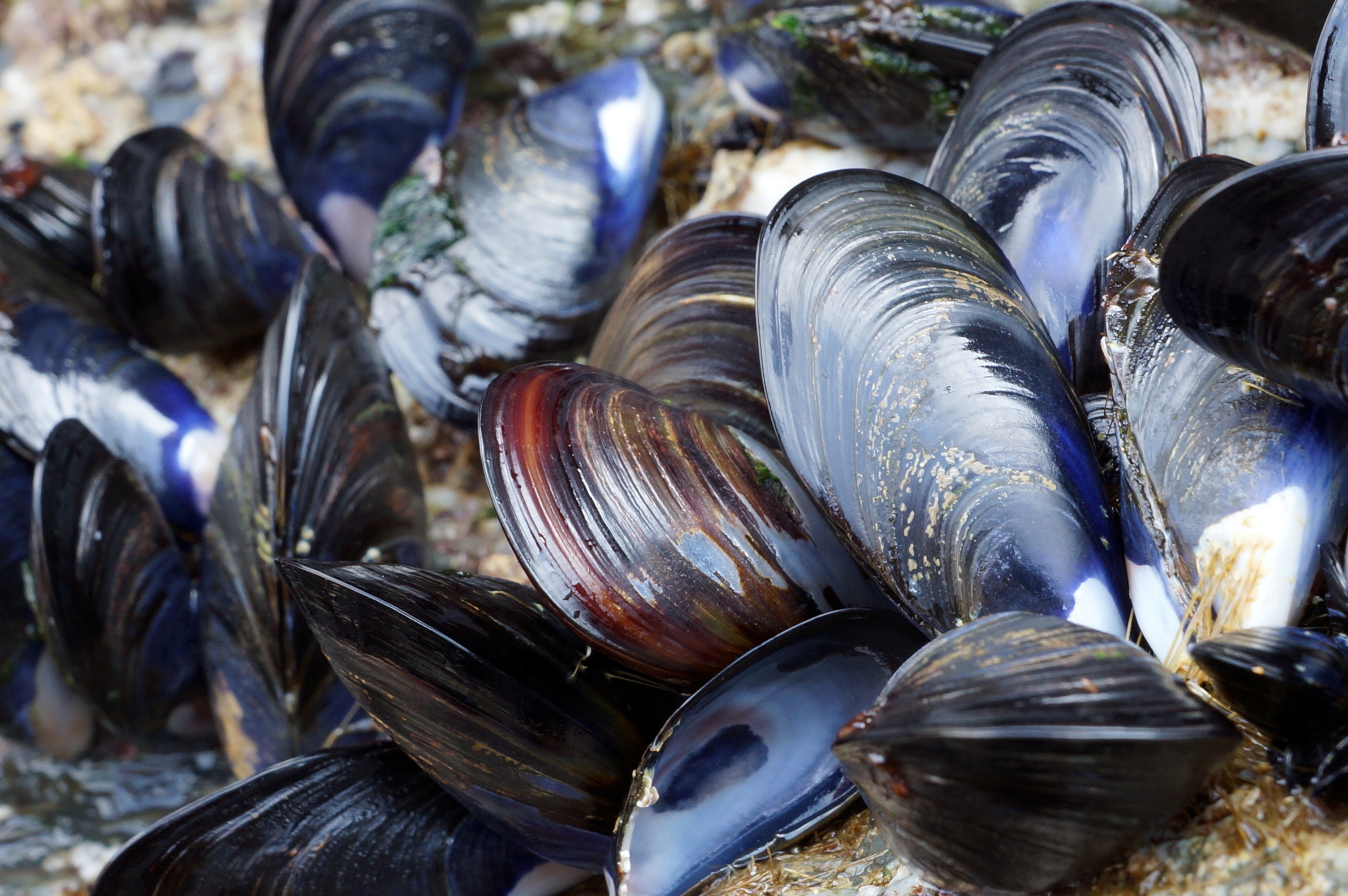
Mercury was found to be problematic in all areas for mussels. © Shutterstock
The OSPAR Hazardous Substances Thematic Assessment is based on measurements of metals in fish, shellfish and sediment collected between 1979 and 2020 from monitoring sites throughout the Arctic Waters, Greater North Sea, Celtic Seas and Bay of Biscay and Iberian Coast Regions, with additional data from marine mammals and birds in the Arctic Waters and some birds in the Greater North Sea. Cadmium and lead concentrations in fish and shellfish were found to be statistically significantly below the background concentration in the Irish and Scottish West Coast, Celtic Seas and the Bay of Biscay and Iberian Coast, whereas lead was below the background concentration in the Irish and Scottish West Coast, Greenland-Scotland Ridge and Norwegian Sea. For mercury in fish and shellfish, no decreasing trends were observed for any sub-regions. On the contrary, increasing trends of 2 to 4% yearly change were observed in the Southern North Sea, the Channel and the Northern Bay of Biscay. Samples taken from sediment showed decreasing trends for mercury in both the Northern and Southern North Sea, the Irish Sea and the Irish and Scottish West Coast, with approximately - 3% yearly change. The increasing trend for fish and shellfish in the Southern North Sea is mainly due to increasing levels in the Wadden Sea area, the Channel, and the Northern Bay of Biscay, mainly along the coast of France. For the Northern Bay of Biscay, no sediment data were available for estimating temporal trends in mercury contamination.
In summary, mercury was found to be problematic in all areas for shellfish and fish, being above both the Background Assessment Concentration (BAC) for mussels and the Quality Standard (QSsp) assessment criteria. Lead and cadmium were above background levels for shellfish and fish in most areas, except the Irish and Scottish West Coast. The Celtic Seas, Iberian Sea and Northern Bay of Biscay were above background levels for cadmium and the Norwegian Sea and Greenland-Scotland ridge for lead. In sediments, mercury and lead were above the Effects Range Low (ERL) assessment criteria in the Southern North Sea, the Channel, the Irish Sea and the Celtic Seas. For the Irish and Scottish West Coast, background levels were achieved in sediments.
Extraction of, or mortality/injury to, wild species (by commercial and recreational fishing and other activities) [Biological]:
The commercial exploitation of fish and shellfish stocks can lead to reduced abundance of target and non-target benthic species. Documented examples include declines in scallop stocks (Howarth and Stuart, 2014) and in threatened and declining species and/or habitats such as Ostrea edulis (OSPAR, 2008). The use of mobile fishing gear targeting the queen scallop (Aequipecten opercularis) has caused damage and the eventual collapse of Modiolus beds in Northern Ireland and declines in the wider OSPAR Maritime Area (OSPAR, 2009, Fariñas-Franco et al., 2018). Bottom fishing can lead to an influx of scavengers in recently fished areas, reduce community production, change the size/age structure of benthic species and trigger cascade effects altering the trophic structure and function of benthic habitats (Coleman and Williams, 2002; Sciberras et al., 2018; Fennell et al., 2021). The collection of mussel seed for aquaculture (e.g., poorly managed dredging) can have an impact on wild mussel populations. For example, past seed collection is associated with the declines in the mussel beds of the Wadden Sea (See: OSPAR Feeder Report 2021 - Aquaculture , OSPAR Feeder Report 2021 – Extraction of non-living resources ).
Input or spread of non-indigenous species [Biological]:
Non-indigenous species (NIS) have the potential to outcompete native benthic species, altering community, habitat and ecosystem structures and functions. Genetically modified species and translocation of native species are also part of this biological pressure. New introductions occur in all OSPAR Regions every year, but their impacts on benthic habitats are rarely or only locally monitored and quantified. Climate change may facilitate the successful establishment and spread of several opportunistic NIS which benefit from its negative impacts on native species and communities. More details and references on this pressure and its drivers, impacts and responses are available in the OSPAR Non-indigenous Species Thematic Assessment . (See: OSPAR Indicator Assessment - Trends in New Records of Non-indigenous Species Introduced by Human Activities )
Input of litter (solid waste matter, including micro-sized litter) [Substances, litter, energy]:
Marine litter tends to sink and accumulate through time in benthic habitats (Thushari and Senevirathna, 2020). Marine litter (including plastics) can lead to the smothering of benthic habitats and generation of artificial hard substrate, altering the structure of benthic communities and leading to loss of biodiversity. Marine animals can ingest or become entangled in litter on or near the seafloor, resulting in death or injury, OSPAR Indicator Assessment - Composition and Spatial Distribution of Litter on the Seafloor ; Consoli et al., 2019). Plastic items are highly likely to degrade to micro-sized plastics that have a higher potential to accumulate on benthic biota, with adverse effects on survival and reproduction (Thushari and Senevirathna, 2020). Plastic items are potential sources of contaminants because of their chemical additives and because they can concentrate contaminants already present in the water column (Chen et al., 2019); they can also cause habitat damage or act as a transport vector for invasive species (García-Gómez et al., 2021). Plastic items related to fishing are reported as one of the most common forms of litter in the marine environment ( OSPAR Intermediate Assessment 2017 , Kammann et al., 2018). Abandoned, lost or discarded fishing gear may represent one of the main causes of degradation of benthic habitats (Galgani et al., 2018; Gilman et al., 2021). (See: Marine Litter Thematic Assessment )
Input of other forms of energy (including electromagnetic fields, light and heat) [Substances, litter and energy]:
Heat, light, sound and electromagnetic fields can cause alterations to environmental conditions, and therefore have an adverse effect on benthic organisms.
Thermal input:
Thermal power plants require large inputs of water for cooling; consequently, they are often constructed near coastal areas. Seawater in most cases is pumped into the power plants and subsequently ejected back into the environment as heated thermal effluent (Poornima et al., 2005). The impacts include reduction of biomass in the general vicinity of the discharge location, increases in invasive species, alteration of substrate type, community alteration and negative effects on recruitment (Kramer, 1994; Chou et al., 2004).
Changes in current flow:
In suitable coastal locations, generators are built to convert the energy from the rise and fall of tides into electrical power. These generators can alter flow dynamics and sediment transport characteristics, leading to faunal changes (Baker et al., 2020). The impact on benthic assemblages can occur at some distance from where the energy is produced, both for benthic individuals and pelagic larval stages.
Electromagnetic fields:
Interaction with electromagnetic fields (EMF), for example from offshore wind infrastructure, has the potential to induce behavioural, physiological and developmental effects in benthic and demersal biota. For example, altered sheltering behaviour and increased exploratory response have been observed in the American lobster (Homarus americanus) when exposed to high voltage EMF. Demersal and benthic species (e.g., certain elasmobranchs) can also be influenced by EMF and can show increased exploratory and foraging behaviour, thus increasing the predation on benthic fauna (Hutchison et al., 2020)
Extraction of, or mortality/injury to, wild species (by commercial and recreational fishing and other activities) [Biological]:
Coleman, F.C. and Williams, S.L., 2002. Overexploiting marine ecosystem engineers: potential consequences for biodiversity. Trends in Ecology & Evolution, 17(1), pp.40-44.
Fennell, H., Sciberras, M., Hiddink, J.G., Kaiser, M.J., Gilman, E., Donnan, D. and Crawford, R. 2021. Exploring the relationship between static fishing gear, fishing effort, and benthic biodiversity: a systematic review protocol. Environmental Evidence, 10(1), pp.1-8.
Fariñas-Franco, J.M., Allcock, A.L. and Roberts, D. 2018. Protection alone may not promote natural recovery of biogenic habitats of high biodiversity damaged by mobile fishing gears. Marine Environmental Research, 135, pp.18-28.
Howarth, L.M. and Stewart, B.D., 2014. The dredge fishery for scallops in the United Kingdom (UK): effects on marine ecosystems and proposals for future management.
OSPAR Commission, 2009: Background document for Modiolus modiolus. https://www.ospar.org/documents?v=7193
Sciberras, M., Hiddink, J.G., Jennings, S., Szostek, C.L., Hughes, K.M., Kneafsey, B., Clarke, L.J., Ellis, N., Rijnsdorp, A.D., McConnaughey, R.A. and Hilborn, R. 2018. Response of benthic fauna to experimental bottom fishing: A global meta‐analysis. Fish and Fisheries, 19(4), pp.698-715.
Input of litter (solid waste matter, including micro-sized litter) [Substances, litter, energy]:
Chen, Q., Allgeier, A., Yin, D. and Hollert, H., 2019. Leaching of endocrine disrupting chemicals from marine microplastics and mesoplastics under common life stress conditions. Environment international, 130, p.104938.
Consoli, P., Romeo, T., Angiolillo, M., Canese, S., Esposito, V., Salvati, E., Scotti, G., Andaloro, F. and Tunesi, L. 2019. Marine litter from fishery activities in the Western Mediterranean Sea: The impact of entanglement on marine animal forests. Environmental Pollution, 249, pp.472-481.
Galgani, F., Pham, C.K., Claro, F. and Consoli, P. 2018. Marine animal forests as useful indicators of entanglement by marine litter. Marine pollution bulletin, 135, pp.735-738.
García-Gómez, J.C., Garrigós, M. and Garrigós, J. 2021. Plastic as a vector of dispersion for marine species with invasive potential. A review. Frontiers in Ecology and Evolution, 9, p.629756.
Gilman, E., Musyl, M., Suuronen, P., Chaloupka, M., Gorgin, S., Wilson, J. and Kuczenski, B. 2021. Highest risk abandoned, lost and discarded fishing gear. Scientific reports, 11(1), pp.1-11.
Kammann, U., Aust, M.O., Bahl, H. and Lang, T. 2018. Marine litter at the seafloor–Abundance and composition in the North Sea and the Baltic Sea. Marine pollution bulletin, 127, pp.774-780.
Thushari, G.G.N. and Senevirathna, J.D.M. 2020. Plastic pollution in the marine environment. Heliyon, 6(8), p.e04709.
Input of other forms of energy (including electromagnetic fields, light and heat) [Substances, litter and energy]:
Baker, A., Craighead, R.M., Jarvis, E.J., Stenton, H.C., Angeloudis, A., Mackie, L., Avdis, A., Piggott, M.D. and Hill J. (2020) ‘Modelling the impact of tidal range energy on species communities’, Ocean & Coastal Management, Volume 193, 2020, 105221, ISSN 0964-5691, https://doi.org/10.1016/j.ocecoaman.2020.105221
Chou, Y., Lin, T.Y, Chen, C.T.A and Liu, L.L.l. (2004) ‘Effects of Nuclear Power Plant Thermal Effluent on Marine Sessile Invertebrate Communities in Southern Taiwan’, Journal of Marine Science and Technology, 12(5). Available at: https://doi.org/10.51400/2709-6998.2267
Hutchison, Z.L., Secor, D.H. and Gill, A.B., 2020. The interaction between resource species and electromagnetic fields associated with electricity production by offshore wind farms. Oceanography, 33(4), pp.96-107. https://doi.org/10.5670/oceanog.2020.409
Kramer, K.J.M. (1994) Biomonitoring of coastal waters and estuaries. Available at: https://www.vliz.be/en/imis?refid=5677 (Accessed: 13 October 2022)
Poornima, E.H., Rajaduraia,M., Rao, T.S., Anupkumar, B., Rajamohan, R., Narasimhan, S.V., Rao, V.N.R and Venugopalan, V.P. (2005) ‘Impact of thermal discharge from a tropical coastal power plant on phytoplankton’, Journal of Thermal Biology, 30(4), pp. 307–316. Available at: https://doi.org/10.1016/j.jtherbio.2005.01.004
Activities | State |